Biomedical Engineering Theory And Practice/Biomechanics IV
Cardiac Bio-mechanics
[edit | edit source]
The mammalian heart is composed of four pumping chambers: upper left and right atria; and lower left and right ventricles. The atria are the two upper chambers of the heart. The right atrium receives and holds deoxygenated blood from the superior vena cava, inferior vena cava and coronary sinus and then sends down to the right ventricle which in turn sends it to the pulmonary trunk and artery for pulmonary circulation. The left atrium receives the oxygenated blood from the left and right pulmonary veins and then pumps to the left ventricle for pumping out through the aorta for systemic circulation[1][2]The atria do not have valves at their inlets.[3]. In the heart, a ventricle is one of the two lower chambers of the heart[4].The right ventricle receives blood from the right atrium and pumps it into the lungs via the pulmonary artery, and the left ventricle receives blood from the left atrium and pumps it into the circulation system via the aorta[4].
TABLE Basic Determinants of Myocardial Stress and Strain
Geometry and structure | Three-dimensional shape | Wall thickness,Curvature,Stress-free and unloaded reference configurations |
Tissue structure | Muscle fiber architecture,Connective tissue organization,Pericardium, epicardium, and endocardium | |
Boundary/initial conditions | Pressure | Filling pressure (preload),Arterial pressure (afterload),Direct and indirect ventricular interactions |
Constraints | Effects of inspiration and expiration,Constraints due to the pericardium and its attachments,Valves and fibrous valve annuli, chordae tendineae,Great vessels, lungs | |
Material properties | Resting or passive | Nonlinear finite elasticity,quasilinear viscoelasticity,Anisotropy,Biphasic poroelasticity |
Active dynamic | Activation sequence,Myofiber isometric and isotonic contractile dynamics,Sarcomere length and length history,Cellular calcium kinetics and metabolic energy supply |
Source:Bronzino, Joseph D. (April 2006). The Biomedical Engineering Handbook, Third Edition. [CRC Press]. p. 54-2. ISBN 978-0-8493-2124-5.
Table Factors Affected by Myocardial Stress and Strain
Direct factors | Regional muscle work,Myocardial oxygen demand and energetics,Coronary blood flow |
Electrophysiological responses | Action potential duration (QT interval),Repolarization (T wave morphology),Excitability |
Development and morphogenesis | Growth rate,Cardiac looping and septation,Valve formation |
Vulnerability to injury | Ischemia,Arrhythmia,Cell dropout |
Remodeling, repair, and adaptation | Eccentric and concentric hypertrophy,Fibrosis,Scar formation |
Progression of disease | Transition from hypertrophy to failure,Ventricular dilation,Infarct expansion,Response to reperfusion,Aneurysm formation |
Source:Bronzino, Joseph D. (April 2006). The Biomedical Engineering Handbook, Third Edition. [CRC Press]. p. 54-2. ISBN 978-0-8493-2124-5.
Collagen and Heart Wall
[edit | edit source]Collagen
[edit | edit source]Collagen is composed of a triple helix:two identical chains (α1) and an additional chain that differs slightly in its chemical composition (α2).[5]. A collagen fiber has a greater tensile strength than a steel wire of equal size[6][7] .Until now, 28 types of collagen have been identified and described. The five common types are:
- Collagen I[8]:This is the most plentiful collagen of the human body.It is found in skin, tendon, vascular ligature, organs, bone (main component of the organic part of bone)
- Collagen II[9]: It makes up 50% of all protein in cartilage and 85-90% of collagen of articular cartilage.Type II collagen does form fibrils. This fibrillar network of collagen entrap the proteoglycan aggregate as well as provide tensile strength to the tissue.
- Collagen III[10]: Collagen alpha-1(III) chain is a fibrillar collagen that is found in extensible connective tissues such as skin, lung, and the vascular system, frequently with type I collagen.
- Collagen IV: It forms basal lamina, the epithelium-secreted layer of the basement membrane.
- Collagen V: It is found in cell surfaces, hair and placenta
Heart Wall
[edit | edit source]
The myocardium is composed of myocytes, extracellular matrix, and the capillaries. While only consisting of 1-4% of total heart protein, Type I and III collagen is essential to maintain the size and shape of the heart. The collagen matrix combines myoctyes, myofilaments, and the capillaries to distribute force along the ventricle walls. The cardiac extracellular matrix consists primarily of the fibrillar collagens, type I (85%) and III (11%), synthesized by the cardiac fibroblasts.
Collagen content, organization, cross-linking and ratio of types I to III change with age and in various disease conditions including myocardial ischemia and infarction, hypertension and hypertrophy[11][12][13]. After a myocardial infarction, the composition of collagen in the myocardial muscle changes. It increases from 40% to 150% during hypertension and ventricular pressure overload. Hypertrophy is from high increases of collagen Type III in the myocardial muscle[14]. Fibrillar collagen is also a major component in the scar after an MI. During ventricular dilation, a decrease in collagen cross-linking is observed because of the replacement of mature collagen with new collagen with decreased cross-linking.Collagen intermolecular cross-linking is controlled by two separate mechanisms. The formation of enzymatic hydroxylysyl pyridinoline cross-links is catalyzed by lysyl oxidase, which needs copper as a catalyst. Nonenzymatic collagen cross-links known as advanced glycation endproducts can be formed because of reducing sugars[15][16]. Since the collagen is important to maintain myocyte shape and alignment, this weakness cause ventricular dilation and systolic dysfunction.
Ventricular Geometry
[edit | edit source]
In view of engineering mechanics, the ventricles are three-dimensional thick-walled pressure vessels with change of wall thickness and principal curvatures both locally and temporally through the cardiac cycle. Ventricles have thicker walls than atria as it should generate higher blood pressures. The ventricular walls in the normal heart are thickest at the equator and base of the left ventricle which needs to pump blood to most of the body while the right ventricle fills only the lungs. The ventricular walls in the normal heart are thinnest at the left ventricular apex and right ventricular free wall. The physiologic load on the ventricles requiring pumping of blood throughout the body and lungs is much greater than the pressure by the atria to fill the ventricles. The mass of the left ventricle, according to magnetic resonance imaging, averages 143 g ± 38.4 g, with a range of 87–224 g.[17] The right ventricle is equal in size to the left ventricle, and contains about 85 millilitres (3 imp fl oz; 3 US fl oz) in the adult.
Myofiber Architecture
[edit | edit source]

The cardiac ventricles have a complicated three-dimensional muscle fiber architecture[18]. The myofibers are composed of myofibrils. The myofibrils consist of actin and myosin filaments repeated in units called a sarcomere, the basic functional unit of the muscle fiber. The sarcomere is responsible for skeletal muscle's striated appearance and muscle contraction. In the heart of various mammals, although the myocytes are relatively short, they are connected with orientation which is commonly quantified by helix and transverse angles[19][20][21][22]. The helix angle represents the longitudinal component of the fiber orientation, whereas the transverse angle represents the transmural component of the fiber orientation. Measured transmural helix angle is typically range from +60° at the subendocardium to −60° at the subepicardium, although it is various according to measurements. And according to mathematical models of cardiac wall mechanics, it is considered that the distribution of myofiber orientation within the cardiac wall determine the distribution of stress and myofiber shortening throughout the wall during ejection[23][24][25][26].
While the most trustable results of ventricular myofiber architecture have been done through quantitative histological techniques, diffusion tensor magnetic resonance imaging (MRI) has proven to be a reliable technique for estimating fiber orientation nondestructively in fixed and even intact beating human hearts[27][28][29]. And according to David E et al.(2009), diffusion spectrum MRI tractography (DSI tractography), was used to image myofiber architecture in normal and infarcted myocardium. Unlike diffusion tensor imaging, DSI tractography resolves multiple myofiber populations per voxel and generates accurate 3D tractograms present in the myocardium for the first time[30].
Cardiac Pump Function
[edit | edit source]Cardiac Cycle
[edit | edit source]

The most basic mechanical parameters of the cardiac pump are blood pressure and volume flowrate, specially in the major pumping chambers, the ventricles. In view of wall mechanics, the ventricular pressure is essential boundary condition. Cardiac cycle event related to pressure and volume in the left ventricle are shown in Figure. Stages of the cardiac cycle is divided into five steps: [31]
- Atrial Systole:Mitral valve opens rapidly and semilunar valves is closed.Atria contract and pump blood.Ventricles, already partially filled from phase 5, receive last ~30% of blood, for a final resting volume of about 130mL.
- Isovolumetric Contraction:The deceleration of the inflowing blood reverses the pressure across the valve leaflets and causes them to close mital valve closure and semilunar valves is still closed. Ventricles begin to contract. Ventricular muscle initially shortens a little, but intraventricular pressure rises rapidly(about 50 msec in adult humans). Ventricular volume unchanged.
- Ventricular Ejection:Atrioventricular valves close but semilunar valves open. Pressures in left and right Ventricle exceed pressures in Aorta (80mmHg) and Pulmonary Artery (10mmHg). Ejection is quick at first, slowing down as systole progresses.At Amount ejected each ventricle per stroke at rest is 70-90mL. About 50mL of blood remains in each ventricle at the end of systole
- Isovolumetric Relaxation:All the valves close as Ventricles relax and pressure within Ventricles drops below 120mmHg. This ends once Ventricular Pressure falls below Atrial pressure until Atrioventricular valves open. The heart pump blood to rest of body.
- Ventricular Filling:Atrioventricular valves open and semilunar valve close. Ventricles is relaxed.Ventricles passively fill with approximately 70% of their final volume. As the ventricles fill, rate of filling decreases and the AV valves drift towards closing.Atria expand and are filling.
The phases of the cardiac cycle are customarily divided into two: systole and diastole. The end of diastole-the start of systole — is generally defined as the time when mitral valve opens rapidly and semilunar valves is closed.
Ventricular Pressure-volume relationship
[edit | edit source]
Real-time left ventricular (LV) pressure-volume loops shows the relationship between ventricular pressure and volume changes effectively. During the last 20 years, the ventricular pressure–volume relationship has been studied extensively, specially by Sagawa, and colleagues[32].The isovolumic phases of the cardiac cycle can be the vertical segments of the loop, the lower limb is the ventricular filling, and the upper segment is the ejection phase. Changes in the filling pressure of the ventricle (preload) move the end-diastolic point along the unique end-diastolic pressure–volume relation (EDPVR), which means the passive filling curve for the ventricle and thus the passive properties of the myocardium. The locus of end-ejection points (AVC) forms the end-systolic pressure–volume relation (ESPVR), which describes the maximal pressure that can be developed by the ventricle at any given LV volume. It is linear in various condition and mostly independent of changes in preload, afterload, and heart rate. The difference between the end-diastolic volume (EDV) and the end-systolic volume (ESV) is the stroke volume, which is the volume of blood ejected by the right/left ventricle in a single contraction. In mathematical terms, SV = EDV – ESV.
The area of the ventricular pressure–volume loop is a measure of the ventricular stroke work performed by the myocardium on the ejecting blood.It is a product of the stroke volume and the mean aortic or pulmonary artery pressure (afterload), relying on whether one is considering the left or the right ventricle.
Cardiac Muscle Contraction
[edit | edit source]
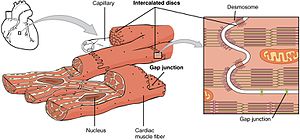

Cardiac muscle cells and skeletal muscle cells have similar, but not identical in contractile mechanisms. Both striated muscle cell (cardiac and skeletal) is composed of smaller units called myofibrils, which in turn are made up of sarcomeres. The sarcomere contains overlapping thin and thick filaments, which are responsible for the force development in the muscle cells.The ability of a muscle to produce tension depends on the overlap between thick and thin filaments. Compared to skeletal muscle, cardiac muscle needs extracellular calcium ions for contraction to occur.
Table Basic mechanical variables in striated muscle contraction.
Isometric tension ( ) | the tension is generated by a muscle contracting at a fixed length. The maximum isometric tension (for a maximally activated muscle) is about constant for skeletal muscle, but for cardiac muscle depends on length. |
Tension ( ) | Actively developed tension. Usually a function of isometric tension and the rate of shortening:
,where V is the rate of shortening and f(V) is some force-velocity relation. |
Fibre extension ratio ( λ ) | Current sarcomere length divided by the slack length. |
Archibald Vivian Hill(1938)[33] describes the relation between velocity and tension in a muscle that contracts against a constant load (isotonic contraction). , where is the isometric tension and V is the velocity. a and b are parameters fitted to experimental data. is constant for skeletal muscle cells, but depends on length in cardiac cells. But, the Hill function only includes the current velocity.
In fact, for the detailed mathematical model for the actively contracting muscle fiber should contain the following:
- Half maximal tension in cardiac muscle is developed at intracellular calcium concentrations of 10−6 to 10−5, M depending on factors such as species and temperature [34].
- The concentration of calcium bound to Troponin C which depends on and the tension T .
- The proportion of actin sites available for cross-bridge binding which depends on the concentration of calcium bound to Troponin C[35].
- The length-tension relation and Force-velocity dependence.
Therefore, relative isometric tension max may be modeled using [36][37]:
The Hunter-McCulloch-terKeurs (HMT) model[37] used the following assumptions to fit the data of Kentish et al.[38] from rat right ventricular trabeculae:
where the reference sarcomere length was taken to be 2.0 μm.The HMT model uses a standard Hill function, but with velocity V replaced by a so-called fading memory model, which contains information on the history of length changes. Coupling of the HMT model to an lectrophysiology model is acceptable because of the dependence on intracellular calcium. For the reality of the coupled model the cell model should contain stretch-activated channels. This permits a two-way coupling between the electrophysiology and the mechanics of the muscle, excitation-contraction coupling and mechano-electric feedback. The HMT model only provides the information about a single muscle fibre. The deformation of the muscle is the result of active force developed in the cells, and passive forces developed by the elastic properties of the tissue. For modeling the deformation of the muscle tissue needs advanced continuum mechanics.
Resting Myocardial properties:Stress and Strain
[edit | edit source]
The circulatory system is composed of two circulations (pulmonary and systemic) in series between the right ventricle (RV) and left ventricle (LV). Balance is achieved mainly by the Frank-Starling mechanism.
Heart Valve Dynamics
[edit | edit source]The heart valves allow blood to flow in only one direction through the heart, permitting forward flow and preventing back flow. Commonly, there are four valves in our human mammalian heart. A heart valve opens or closes depending on different blood pressure on each side.[39][40][41] In the right heart,the tricuspid and pulmonic valves control the blood flow returned from the body to the lungs for oxygenation. The mitral and aortic valves regulate the flow of oxygenated blood from the left side of the heart to the body. The aortic and pulmonic valves are located at the base of the aorta and the pulmonary trunk or artery of the left and right sides of the heart, respectively. The mitral and tricuspid valves are located in the atria and ventricles of the left and right sides of the heart, respectively. The aortic and pulmonic valves open during systole when the ventricles are contracting, and close during diastole when the ventricles are filling through the open mitral and tricuspid valves. During isovolumic contraction and relaxation, all four valves are closed.
Semilunar Valves
[edit | edit source]Structure of Semilunar Valves
[edit | edit source]
The two semilunar (SL) valves, the aortic valve and the pulmonary valve, which are placed at the base of the aorta and the pulmonary trunk or artery, and the aorta. These two arteries receive blood from the ventricles and their semilunar valves allow blood to be forced into the arteries, and prevent backflow from the arteries into the ventricles. These valves have no chordae tendineae, and are similar to the valves in veins than the atrioventricular valves.The second heart sound is due to closure of the semilunar valves.

The aortic valve is composed of three semilunar cusps, or leaflets , included within a connective tissue sleeve.The cusp is a complicated and multi-layer with 300~700µm thickness. In cross-section, the cusp has three distinct layers, the fibrosa(~45%), spongiosa(~35%) and ventricularis(~20%). Each of the cusps is lined with endothelial cells and has a dense collagenous core adjacent to the high pressure aortic side. The ventricularis covers the ventricular side of the valve and consists of collagen and elastin. The central part,called the spongiosa, includes variable loose connective tissue and proteins. The collagen fibers within the fibrosa and ventricularis are unorganized in the unstressed state. When a stress is applied, they become oriented mainly in the circumferential direction with a lower concentration of elastin and collagen in the radial direction[42][43].The anatomy of the pulmonic valve is similar to that of the aortic valve, but the surrounding structure is a little bit different. There are various method for the studies.According to an pathological experiments, the aortic valve diameter was 23.2 ± 3.3 mm, whereas the diameter of the pulmonic valve was measured at 24.3 ± 3.0 mm [44]. But, according to M-mode echocardiographic study, the aortic root diameter at end systole was 35 ± 4.2 mm and 33.7 ± 4.4 mm at the end of diastole[45].
Dynamics and mechanics of Semilunar Valves
[edit | edit source]Systolic contraction of the left ventricle forces the aortic valve leaflets to open, allowing oxygenated blood to enter the aorta. The reversed pressure gradient, created when the heart rests in diastole, causes the aortic valve leaflets to close.
Atrioventricular Valves
[edit | edit source]Structure of atrioventricular Valves
[edit | edit source]
These are the mitral and tricuspid valves located between the atria and the ventricles that prevent backflow from the ventricles into the atria during systole.The mitral and tricuspid valves are similar in structure as both valves consist of four primary elements (1) the valve annulus, (2) the valve leaflets, (3) the papillary muscles, and (4) the chordae tendineae. On the left side, is the two-piece mitral valve. The mitral annulus is an elliptical ring composed of dense collagenous tissue surrounded by muscle and attached to the mitral valve leaflets.The mitral annulus changes the size and the shape three-dimensionally and dynamically during the cardiac cycle.The normal diameter of the mitral annulus is 3.1 ± 0.4 cm, and the circumference is from 8 to 12 cm during diastole[46][47][48]. It is smaller at the end of atrial systole because of the contraction of the left atrium around it, like a sphincter. The mitral valve is a bileaflet valve composed of an anterior and posterior leaflet. The leaflet tissue consists of mainly collagen-reinforced endothelium and a little bit striated muscle cells, non-myelinated nerve fibers, blood vessels. The anterior and posterior leaflets of the valve are one continuous piece of tissue and separated by commisures.The location of the commisures can be identified by chordae tendinae. The total surface area of both leaflets is about twice of the mitral orifice;This extra surface area helps mitral annulus shape change during the cardiac cycle. The anterior leaflet protects about two-thirds of the valve and is divided into three scallop (1) the medial, (2) central, and (3) lateral scallop [49][50][51][52][53]. Although the anterior leaflet takes up a larger part of the ring, the posterior leaflet has a larger surface area. These valve leaflets are kept from prolapsing into the left atrium by the action of chordae tendineae, the tendon attached to posterior surface of the valve.Leaking valves can be corrected by mitral valve annuloplasty

The mitral leaflet tissue can be classified into both a rough and clear zone.Unlike the mitral valve, The right side atrioventricular ring, the tricuspid valve has three leaflets (1) an anterior leaflet, (2) a posterior leaflet with a variable number of scallops, and (3) a septal leaflet.The tricuspid valve is larger and tructurally more complicated than the mitral valve.
The chordae tendineae (tendinous chords), or heart strings, are cord-like tendons that connect the papillary muscles to both leaflets in the heart. Chordae tendineae are about 80% collagen and the remaining 20% composed of elastin and endothelial cells. The chordae tendineae is made up of an inner core of collagen surrounded by loosely meshed elastin and collagen fibers with an outer layer of endothelial cells. From each papillary muscle, several chordae originate and branch into the marginal and basal chordae. The thinner marginal chordae insert into the leaflet free edge at .But the thicker basal chordae insert into the leaflets at a higher level towards the annulus. The marginal chordae keep the leaflets stationary while the basal chordae act more as supports[54]. There are five total papillary muscles in the heart, three in the right, tricuspid valve and two in the left,mitral valve. The papillary muscles of both ventricles start to contract shortly before ventricular systole and maintain tension throughout[55][56].
Dynamics and mechanics of atrioventricular Valves
[edit | edit source]Mechanics of Blood Vessels
[edit | edit source]
The blood vessels are the part of the circulatory system that transports blood throughout the human body[57]. There are three major types of blood vessels: the arteries, which carry the blood away from the heart; the capillaries, which exchange water and chemicals between the blood and the tissues; and the veins, which carry blood from the capillaries back toward the heart[57].
The vascular wall anatomy
[edit | edit source]Layered microstructure of the vascular wall
[edit | edit source]Blood vessels consist of three layers:the intima, media, and adventitia.
- Tunica intima(the thinnest single layer): It is composed of one layer of endothelial cells and is supported by an internal elastic lamina.They are involved in control of vessel diameter.
- Tunica media(the thickest layer in arteries) :It is composed of elastin, collagen, and smooth muscle, largely determines the elastic properties of the vessel.
- Tunica adventitia:It consists mainly of connective tissue. It is mainly composed of collagen and, in arteries, is supported by external elastic lamina. It also includes nerves that supply the vessel as well as nutrient capillaries (vasa vasorum) in the larger blood vessels.
Structural elements of vascular wall
[edit | edit source]Mechanical Characteristics of the vascular wall
[edit | edit source]Blood vessels, similar to the other soft biological tissues, do not obey Hooke’s law, becoming stiffer as pressure is increased. They also show viscoelastic properties like hysteresis and creep.
Elasticity of the vascular wall
[edit | edit source]Arterial Hemodynamics
[edit | edit source]Arterial Anatomy
[edit | edit source]

Blood Flow
[edit | edit source]
Blood consists of blood cells suspended in blood plasma. A nearly Newtonian fluid,plasma contains 91.5% water, 7% proteins and 1.5% glucose, mineral ions, hormones, carbon dioxide and so on[58].The blood cells are mainly red blood cells (also called RBCs or erythrocytes) and white blood cells, including leukocytes and platelets. In very smaller vessel(about 100 μm) shows clear non-Newtonian effects. But flow in thicker vessels shows the Newtonian assumptions is acceptable[59][60].
Blood flow in the artery is mostly laminar with the exception of the proximal aorta and main pulmonary artery. The blood flow velocity is the fastest in the middle of the vessel and slowest at the vessel wall. Mostly, the mean velocity is used[61].
Wave Propagation
[edit | edit source]
The viscoelasticity of blood vessels affects the arterial flow. Pulse wave velocity (PWV) is a measure of arterial stiffness. [62] [63] It is easy to measure invasively and non-invasively in humans and is highly reproducible.[64]
The Moens–Korteweg equation states that PWV is proportional to the square root of the incremental elastic modulus, (Einc), of the vessel wall given constant ratio of wall thickness, h, to vessel radius, r, and blood density, ρ, assuming that the artery wall is isotropic and experiences isovolumetric change with pulse pressure.[65]
Pathology
[edit | edit source]
Atherosclerosis (also known as arteriosclerotic vascular disease or ASVD) is a disease in which an artery wall thickens because of invasion and accumulation of white blood cells (WBCs).The disease starts with a thickening of the intimal layer in locations related to the shear stress distribution on the endothelial surface[66]. Plaque (plak) which builds up inside your arteries, is composed of fat, cholesterol, calcium, and other substances found in the blood. Over time, plaque hardens and narrows your arteries. This limits the flow of oxygen-rich blood to your organs and other parts of your body.The disease is dangerous in heart, brain, arms, legs, pelvis, and kidneys. Atherosclerosis can cause to serious problems, including heart attack, stroke, or even death. Including intimal thickening, the arterial wall properties also depends on age.
The Venous Return
[edit | edit source]
Venous return is the rate of blood flow back to the heart. Under steady-state conditions, venous return should be equal to cardiac output (Q), when averaged over time as the cardiovascular system is a closed loop. Otherwise, blood would accumulate in either the systemic or pulmonary circulations. The circulatory system is composed of two circulations (pulmonary and systemic) situated in series between the right ventricle (RV) and left ventricle (LV). Balance is achieved, in large part, by the Frank–Starling mechanism. Hemodynamically, venous return (VR) to the heart from the venous vascular beds is estimated by a pressure gradient (venous pressure - right atrial pressure) and venous resistance (RV)[67].
Cardiac Output
[edit | edit source]Cardiac output (Q or or CO ) is the amount of blood the heart pumps through the circulatory system in a minute. The amount of blood put out by the left ventricle of the heart in one contraction is called the stroke volume. The stroke volume and the heart rate determine the cardiac output. An average resting cardiac output (Q) would be 5.6 L/min for a human male and 4.9 L/min for a female.[68]
Q = Stroke Volume × Heart rate
Several direct and indirect methods of cardiac output measurement are available. Cardiac output measurement should be accurate, precise, operator independent, fast responding, non-invasive, continuous, easy to use, cheap and safe. This method does not exist today.
Fick's cardiac output measurement
[edit | edit source]Direct Fick for oxygen [Fick O2]
[edit | edit source]In 1870, Adolf Fick described a method to calculate cardiac output based on a mass balance for oxygen. In this method, the following variables are measured:[69]
- VO2, oxygen consumption in ml of pure gaseous oxygen per minute. This may be measured by a spirometer within a closed rebreathing circuit incorporating a carbon dioxide absorber
- Ca, the oxygen concentration of blood from the pulmonary vein (representing oxygenated blood)[70]
- Cv, the oxygen concentration of blood from an intravenous cannula (representing deoxygenated blood)
From this value,
where CO = Cardiac Output, Ca and Cv are the oxygen content of arterial and venous blood, respectively. This allows us to say
and hence calculate cardiac output. (Ca – Cv) is also known as the arteriovenous oxygen difference.[71]
Partial carbon dioxide rebreathing [Fick CO2]
[edit | edit source]The Fick principle can be useful for all gasses that follow Henry's law and diffuse through the lungs, epecially carbon dioxide. The NICO (Novametrix Medical Systems Inc. Wallingford, CT, USA) is studied cardiac output monitor based on the Fick principle for CO2 and uses intermittent partial rebreathing of CO2. Fick's equation applied to carbon dioxide is
where VCO2 is the CO2 production, CaCO2 and CvCO2 the arterial and mixed venous CO2 content in blood.
Dilution Method
[edit | edit source]Today four kinds of the indicator dilution technique are commercially available, i.e. the pulmonary artery catheter thermodilution method with bolus injection of cold fluid, the PAC continuous thermodilution method, the transpulmonary bolus thermodilution method and the transpulmonary lithium bolus dilution method. All these methods is based on a mass balance:
where mi is the amount of indicator injected, q(t) is instantaneous blood flow and c(t) is concentration as function of time.The method measures the concentration of a dye at different points in the circulation, usually from an intravenous injection and then at a downstream sampling site, usually in a systemic artery. If we assume blood flow to be constant, we could find the well-known Stewart-Hamilton equation:
Errors are mainly related to violation of the assumption, inaccurate implementation of the method[72] and anatomic abnormalities[73].
Intermittent pulmonary thermodilution [PAC Td bolus]
[edit | edit source]Since the introduction of the pulmonary artery catheter (PAC) with a thermistor by Swan & Ganz in 1970[74], the thermodilution method has become the standard method to measure cardiac output in patients. The thermodilution method depends on the law of conservation of thermal energy. With temperature as indicator the Stewart-Hamilton equation can be rewritten as follows:
where COtd is cardiac output by thermodilution, Tb is the temperature of blood in the pulmonary artery before injection of injectate, Ti the temperature of the injectate, and ∫ΔTb(t)dt the area under the dilution curve.
PAC continuous cardiac output [PAC CCO]
[edit | edit source]
The Vigilance system (Edwards Lifescience, Irvine, CA, USA) use heat-dilution principles and stochastic system identification together to measure cardiac output[75]. In brief, these methods involve the release of small amounts of heat from a 10-cm thermal filament transported directly into the right ventricular portion of a PAC. Commercially available CCO PACs use different algorithms to analyze the thermal signal measured by the thermistor at the tip of the catheter to arrive at an average cardiac output value. Usually, the displayed value for cardiac output is updated every 30 seconds and represents the average cardiac output over the previous 3 to 6 minutes. Consequently, current thermal signal-based CCO methods should not really be considered continuous, real-time monitors, but rather techniques that provide continual, frequently updated cardiac output values. The PAC CCO monitoring technique is growing in clinical popularity. It is more precise and reproducible than bolus thermodilution cardiac output measurement, but time delaysin the CCO technique need the clinician to depend on other monitored variables to detect acute circulatory changes As an external system for cold fluid injection is not required, the CCO technique needs less nursing time. It may result in fewer measurement errors, less risk of fluid overload, and less risk of infection. This new method needs a significant warm-up time and may work poorly in an environment where there is a great deal of thermal noise, such as the cardiac operating room[75][76].
Intermittent transpulmonary thermodilution [TP Td bolus]
[edit | edit source]This intermittent thermodilution technique nvolves a certain amount of cold fluid is incorporated into the blood stream near the entrance of the right atrium and the dilution curve is detected in the femoral artery[77][78][79].CO is computed with the Stewart-Hamilton equation like the intermittent pulmonary thermodilution technique. In theory, the transpulmonary thermodilution technique should be less accurate because of unpredictable loss of indicator over the lungs, but more precise than pulmonary thermodilution[80][81] as the respiration cycle does not bother the dilution curve. However,the signal-to-noise ratio of the dilution curve decreased.
Transpulmonary lithium dilution [TP Li bolus]
[edit | edit source]The lithium dilution method depends on the venous bolus injection of 1–2 ml of an isotonic lithium chloride solution (150–300 mmol). The resulting arterial lithium concentration–time curve is measured by a lithium sensor in a pre-existing peripheral arterial line. Cardiac output is calculated by the Stewart-Hamilton equation.
where Li, dose is amount of lithium injected, ∫Δc,li(t)dt the area under the lithium dilution curve and PCV the packed cell volume (calculated as the haemoglobin concentration (g dl−1) divided by 34). This correction is necessary as lithium is only diluted in the plasma and not in the red and white cells of blood[82][83]. No side effects have been reported. To be accurate with this technique, the results of three measurements should be done[84]. The lithium dilution method is merged in the LiDCO system (LiDCO, London, UK). The technique is not licensed for the patients weighing less than 40 kg. In addition, the lithium dilution technique should be considered in patients receiving high doses of neuromuscular blocking agents, because of interference with the sensing electrode.
Pulse contour cardiac output
[edit | edit source]
Pulse Pressure (PP) methods measure the pressure in an artery over time to derive a waveform and use this to calculate cardiac output. However any measure from the artery contains the changes in pressure related to changes in arterial function (compliance, impedance and so on). But, it is easy to use. The pulse contour method for estimation of beat-to-beat stroke volume is based on the classical Windkessel model described by Otto Frank in 1899 [85]. At present, four commercial pulse contour cardiac output computers are available: PiCCO, PRAM, LidCO, Vigileo and Modelflow.
Calibrated PP-The PiCCO system [PiCCO] and the LiDCO's pulsco system [LiDCO]
[edit | edit source]PiCCO (PULSION Medical Systems AG, Munich, Germany) and PulseCO (LiDCO Ltd, London, England) make continuous Q by analysis of the arterial PP waveform. In both cases, an independent technique is necessary to calibrate the continuous Q analysis, because arterial PP analysis cannot account for unmeasured variables such as the changing compliance of the vascular bed. Recalibration is needed after changes in patient position, therapy or condition.
The PiCCO algorithm is summarized in the following equation.
where COpi cardiac output, K calibration factor, HR heart rate, P arterial blood pressure, ∫P(t)dt area under the systolic part of the pressure curve, SVR systemic vascular resistance, Cp pressure dependent arterial compliance and dP/dt expresses the shape of the pressure wave.The calibration factor (K) is measured with transpulmonary thermodilution. Recalibration is necessary after profound changes in SVR and at regular (≥1 h) intervals[86][87][88]. Invasive catheterization is needed. For the PiCCO device,the radial and the femoral artery approach can be used[89].
In the case of LiDCO, the independent calibration technique is lithium chloride dilution using the Stewart-Hamilton principle. Lithium chloride dilution uses a peripheral vein to a peripheral arterial line. Like PiCCO, frequent calibration is necessary after a change in Q.Recent data suggest recalibration every 8 h or whenever major haemodynamic changes occur[84].
The pressure recording analytical method [PRAM]
[edit | edit source]PRAM (Vytech Health, Padova, Italy) is a modification of Wesselings cZ algorithm[90]. PRAM estimates CO from the analysis of the pressure wave profile obtained from an arterial catheter (radial or femoral access). Stroke volume (SV) is proportional to the area under the diastolic part of the arterial pressure wave divided by characteristic impedance (Z). The proportionally factor is obtained by calibration with an independent SV measurement (for instance by intermittent thermodilution). Compared to the other methods, PRAM does not depend on calibration or demographic data. With PRAM, Z is obtained from morphological data of the pressure curve of a whole heart beat[91] and is calculated as Z = (P/t) × K(t). Stroke volume (SV) is therefore followed as:
where A is the area under the systolic part of the pressure curve, P/t is the analytical description of the pressure wave form of pressure (P) with time (t) for each heart beat and K(t) is a factor inversely related to the instantaneous acceleration of the cross sectional area of the aorta. With PRAM, stroke volume is calculated for each beat and CO per beat is then derived by multiplying SV with heart rate of the same beat. CO is described as the mean value of 12 beats. As the internal calibration of PRAM is derived from the morphology of the pressure curve, PRAM did not approach the considered standard methods in stable condition[92] and in various hemodynamic conditions;[93] it can be used to monitor pediatric[94] and mechanically supported[95] patients.
Vigileo/FloTrac system [Vigileo]
[edit | edit source]FloTrac/Vigileo (Edwards Lifesciences LLC, U.S.A.) is an uncalibrated pulse contour analysis using a dedicated pressure sensor (FloTrac) and a monitor to compute stroke volume and cardiac output (Vigileo). It does not need an independent calibration. The cardiac output algorithm is based on the principle that is based on Frank–Starling law of the heart, that aortic pulse pressure (PP) is proportional to stroke volume (SV) and inversely related to aortic compliance. The system obtains the pressure signal from any standard peripheral arterial line. From the arterial pressure the standard deviation (σAP) around mean arterial pressure (MAP) is computed over a 20 s interval. This σAP is multiplied by a vascular tone factor (Khi) to generate stroke volume.Khi incorporates to reflect arterial resistance, and compliance is a multivariate polynomial equation that continuously quantifies arterial compliance and vascular resistance. It is calculated by analyzing the patient's heart rate, standard deviation σAP, mean arterial pressure, pressure dependent arterial compliance by the demographics of the patients with the Langewouters equation[96]. The simplified equation is as follows:
Cardiac output is calculated by multiplying stroke volume with heart rate. The wide use of arterial pressure signal processing lets the FloTrac algorithm highly dependent upon a high-fidelity pressure signal.Therefore, the quality of the pressure monitoring signal through testing for optimal dampening and flushing of the arterial line is important.
Modelflow method [Modelflow]
[edit | edit source]Modelflow (FMS, Amsterdam, the Netherlands) is a three-element Windkessel model of the arterial circulation: characteristic impedance which represents the opposition of the aorta to pulsatile inflow, Windkessel compliance which represents the opposition of the aorta to volume increases, and peripheral resistance which represents the opposition of the vascular beds to the drainage of blood. A more details can be found elsewhere[97][98].
Echo-Doppler ultrasound methods
[edit | edit source]Transoesophageal Doppler [TOD]
[edit | edit source]
Nowadays, the Transoesophageal Doppler (TOD) is widely used ultrasound method for fluid management during surgery with evidence for improved patient outcome,[99][100][101][102][103][104][105][106] and has been recommended by the UK's National Institute for Health and Clinical Excellence (NICE). [107]
An oesophageal Doppler probe is inserted into the patient’s oesophagus, and measures velocity of blood flow in the descending aorta. The monitor then displays a velocity/time waveform, providing information for the Clinician to safely manage the patient’s fluid status during surgery. A small ultra-sound transducer, mounted at the tip of a flexible probe, is orally or nasally positioned in the oesophagus along the descending aorta. Insertion depth is generally 35 to 45 cm for adults, according to the route of insertion (oral vs. nasal). The transducer is pointed towards the aorta by rotation to obtain the optimal aortic velocity signal. The blood flow velocity is calculated with the Doppler equation.
where V is the velocity of blood, Fo is the transmitted frequency, Fd is the change in frequency (Doppler shift), cosθ is the angle between the direction of the ultra-sound beam and blood flow and c is the velocity of ultra-sound in blood.
One limitation is that ODM measures the velocity of blood instead of Q and relies on a nomogram[108] according to patient age, height, and weight to convert the measured velocity into Stroke Volume and Cardiac Output.
Transthoracic Doppler [TTE]
[edit | edit source]Transthoracic Doppler (TTD) is an completely non-invasive method which uses an ultrasound probe positioned in the jugular notch to obtain blood velocity in the outflow of the left ventricle. The method is fundamentally equal to oesophageal Doppler technique. Cardiac output is calculated by measuring the cross sectional area of the aortic valve together with the velocity profile in the outflow track. However, it may be very difficult to identify the aortic root in some subjects. In these cases the outflow over the pulmonary valve may be used. The alignment is affected by operator skill, anatomy and subject movements (for example, during breathing).
Thoracic electrical bioimpedance [Bioimpedance]
[edit | edit source]Electrical bioimpedance was introduced as an inexpensive and non-invasiveness cardiac output method about 50 years ago. A high-frequency alternating electrical current with low amplitude is applied to the thorax via two electrodes. The resulting voltage is measured with two other electrodes, placed in between the current electrodes. The measured changes in bio-impedance are thought to be related to changes in cardiac related blood volume. The change in bioimpedance can be translated into cardiac output.
Overview of different methods to measure cardiac output
[edit | edit source]Table.Overview of characteristics for different methods to measure cardiac output
CO method | Invasiveness | Response | Accuracy | Precision | Limitations |
---|---|---|---|---|---|
Fick O2 | +++ | Intermittent | High | Moderate | Requires a PAC for venous O2 and spirometer or mechanical ventilator. Labour-intensive technique |
Fick CO2 | + | Slow | low | low | Subject must be on ventilator Errors due to shunts |
PAC Td bolus | +++ | Intermittent | High | High | Special precaution during mechanical ventilation Requires a PAC and triplicate measurements |
PAC CCO | +++ | Continuous | Moderate | Moderate | Requires a PAC and triplicate measurements |
TP Td bolus | ++ | Intermittent | High | High | Requires a PAC and triplicate measurements |
TP Li bolus | ++ | Intermittent | Moderate | Moderate | Requires only arterial catheter but needs triplicate measurement for sufficient agreement with reference methods |
PiCCO | ++ | Beat-to-beat | Moderate | Moderate | Requires frequent calibration with independent (other) method |
LiDCO | ++ | Beat-to-beat | Moderate | Moderate | Requires frequent calibration with independent (other) method or lithium indicator method |
Vigileo | ++ | Beat-to-beat | Moderate | High | Needs specific sensor |
Modelflow | ++ | Beat-to-beat | High | High | Needs femoral or radial arterial catheter |
TOD | + | Beat-to-beat | High | low | Requires access to oesophagus through mouth or nose |
TTE | - | Intermittent | Moderate | low | Large inter-operator variability |
Bioimpedance | - | Intermittent | low | low | Artifacts due to anatomic variations, shunt, movement, electrical noise |
Source:Bart F Geerts,Leon P Aarts, and Jos R Jansen. Methods in pharmacology: measurement of cardiac output,Br J Clin Pharmacol. Mar 2011; 71(3): 316–330.
Concepts
[edit | edit source]Capacitance
[edit | edit source]The vascular capacitance describes how volume changes in response to a change in the transmural pressure within the blood vessel at hand. Sympathetic activation of veins decreases venous compliance, increases venomotor tone, central venous pressure and promotes venous return indirectly by raising cardiac output through the Frank-Starling mechanism, which increases the total blood flow through the circulatory system. At very high transmural pressures vessels become stiffer, and the incremental volume change to transmural pressure change is small. As all blood vessels shows these nonlinearities, no single parameter can describe capacitance. Therefore, the pressure-volume relationship should be considered.
Venous Compliance
[edit | edit source]
Vascular compliance (C) is described as the slope of the pressure-volume relationship. This ability of a vessel to distend and increase volume with increasing transmural pressure (inside minus outside pressure) is quantified as blood vessel compliance (C), which is the change in volume (ΔV) divided by the change in pressure (ΔP).
In the figure, the slope is not linear because the blood vessel wall is a heterogeneous tissue. It means compliance decreases at higher pressures and volumes. At lower pressures, the compliance of a vein is about 10 to 20-times greater than an artery.
Venous Capacity
[edit | edit source]Capacity is defined as the amount of blood volume in the blood vessels at a specific distending pressure. It is the sum of the unstressed volume and the stressed volume, .
Mean Circulatory Filling Pressure
[edit | edit source]When the inflow and outflow of an organ are suddenly stopped, and blood volume is redistributed, all pressures within the vasculature are the same. This pressure is Mean circulatory filling pressure (Pmcf). It is influenced by the volume of circulating blood and the smooth muscle tone in the walls of the venous system (which determines the capacity of the system).[109] Sometimes, the arterial pressure is not the same to the venous pressure as flow decrease until zero, as blood must move from the distended arterial vessels to the venous beds during the measurement maneuver, and flow may stop before equilibrium. The Pmcf can be measured experimentally by inducing cardiac arrest briefly in animals. The experimentally measured mean circulatory filling pressure gives a good estimate of Pv (the pressure in the minute venules), for estimating venous stressed volume.
Venous Resistance
[edit | edit source]Vascular resistance is the hindrance to flow that must be overcome to push blood through the circulatory system. The resistance offered by the peripheral circulation is known as the systemic vascular resistance (SVR). The resistance by the vasculature of the lungs is known as the pulmonary vascular resistance (PVR). By definition it is the ratio of the pressure gradient between the entrance of the venous circulation, the capillaries, and the venous outflow divided by the venous flow rate.
where R is the venous resistance, Pc is the capillary pressure, Pra is the right atrial pressure, and Q is the venous flow.
Measurement | Reference Range | ||
---|---|---|---|
dyn·s/cm5 | MPa·s/m3 | mmHg·min/l or HRU/Wood units | |
Systemic vascular resistance | 700–1600[110] | 70–160[111] | 9–20[111] |
Pulmonary vascular resistance | 20–130[110] | 2–13[111] | 0.25–1.6[111] |
Venous Inertance
[edit | edit source]Venous inertance (I_v ) is the opposition to a change in flow rate related to the mass of the bolus of blood that is accelerated or decelerated. The inertance Iv for a cylindrical tube with constant cross-sectional area is I_v= Lρ/A, where L is the length of the vessel, ρ is the density of the blood, and A is the cross-sectional area [112].
Techniques to measure venous characteristics
[edit | edit source]Resistance
[edit | edit source]The systemic vascular resistance can therefore be calculated in units of dyn·s·cm−5 as
where mean arterial pressure is 2/3 of diastolic blood pressure plus 1/3 of systolic blood pressure (or Diastolic + 1/3(Systolic-Diastolic).
The pulmonary vascular resistance can therefore be calculated in units of dyn·s·cm−5 as
where the pressures are measured in units of millimetres of mercury (mmHg) and the cardiac output is measured in units of litres per minute (L/min)[113]
Capacitance
[edit | edit source]Compliance
[edit | edit source]
For compliance, changes in volume are necessary. This is easier than measuring the total blood volume. Plethysmography can be used to measure changes in volume in different parts of the body. It involves blood pressure cuffs or other sensors that are attached to a machine called a plethysmograph.
Gravimetric method
[edit | edit source]Gravimetric method is very simple and useful for the measurement of changes in blood volume. It is assumed that 1 g of blood equals to 1 ml of blood. If the organ can be isolated and weighed with the blood vessels intact, changes in volume can be measured.
Further Reading
[edit | edit source]- Bronzino, Joseph D. (April 2006). The Biomedical Engineering Handbook, Third Edition. [CRC Press]. ISBN 978-0-8493-2124-5.
- Villafane, Carlos, CBET. (June 2009). Biomed: From the Student's Perspective, First Edition. [Techniciansfriend.com]. ISBN 978-1-61539-663-4.
{{cite book}}
: CS1 maint: multiple names: authors list (link) - Information related to biomedical engineering.
Practise
[edit | edit source]Reference
[edit | edit source]- ↑ http://www.ivy-rose.co.uk/HumanBody/Blood/Heart_Structure.php
- ↑ Human heart anatomy diagram. Retrieved on 2010-07-02.
- ↑ http://www.americanheart.org/presenter.jhtml?identifier=4598
- ↑ a b >http://www.medicinenet.com/script/main/art.asp?articlekey=9122
- ↑ Szpak, Paul (2011). "Fish bone chemistry and ultrastructure: implications for taphonomy and stable isotope analysis". Journal of Archaeological Science. 38 (12): 3358–3372. doi:10.1016/j.jas.2011.07.022.
- ↑ Fratzl, P. (2008). Collagen: Structure and Mechanics. New York: Springer. ISBN 0-387-73905-X.
- ↑ Buehler, M. J. (2006). "Nature designs tough collagen: Explaining the nanostructure of collagen fibrils". PNAS. 103 (33): 12285–12290. Bibcode:2006PNAS..10312285B. doi:10.1073/pnas.0603216103. PMC 1567872. PMID 16895989.
- ↑ Junqueira's Basic Histology, P106
- ↑ https://en.wikipedia.org/wiki/Type_II_collagen
- ↑ https://en.wikipedia.org/w/index.php?title=Collagen,_type_III,_alpha_1&redirect=no
- ↑ Harper, J., Harper, E., and Covell, J.W., Collagen characterization in volume-overload- and pressure-overload-induced cardiac hypertrophy in minipigs, Am. J. Physiol., 265, H434–H438,1993.
- ↑ Iimoto, D.S., Covell, J.W., and Harper, E., Increase in crosslinking of type I and type III collagens associated with volume overload hypertropy, Circ. Res., 63, 399–408, 1988.
- ↑ Medugorac, I. and Jacob, R., Characterisation of left ventricular collagen in the rat, Cardiovasc. Res., 17, 15–21, 1983
- ↑ Mukherjee, D. and Sen, S., Collagen phenotypes during development and regression of myocardial hypertrophy in spontaneously hypertensive rats, Circ. Res., 67, 1474–1480, 1990.
- ↑ Janeczko RA, Ramirez F (Oct 1989). "Nucleotide and amino acid sequences of the entire human alpha 1 (III) collagen". Nucleic Acids Res. 17 (16): 6742. doi:10.1093/nar/17.16.6742. PMC 318382. PMID 2780304.
- ↑ Superti-Furga A, Gugler E, Gitzelmann R, Steinmann B (Jun 1988). "Ehlers-Danlos syndrome type IV: a multi-exon deletion in one of the two COL3A1 alleles affecting structure, stability, and processing of type III procollagen". J Biol Chem. 263 (13): 6226–32. PMID 2834369.
{{cite journal}}
: CS1 maint: multiple names: authors list (link) - ↑ Schlosser, Thomas; Pagonidis, Konstantin; Herborn, Christoph U.; Hunold, Peter; Waltering, Kai-Uwe; Lauenstein, Thomas C.; Barkhausen, Jörg (2005). "Assessment of Left Ventricular Parameters Using 16-MDCT and New Software for Endocardial and Epicardial Border Delineation". Am J Roentgenol. 184 (3): 765–773. doi:10.2214/ajr.184.3.01840765.
- ↑ Sosnovik DE, Wang R, Dai G, Reese TG, Wedeen VJ,Diffusion MR tractography of the heart,J Cardiovasc Magn Reson (2009)
- ↑ Streeter DD,Spotnitz HM,Patel DP,Ross J,Sonnenblick EH.(1969) Fiber orientation in the canine left ventricle during diastole and systole. Circ Res 24:339–347
- ↑ Streeter DD, Hanna WT.(1973) Engineering mechanics for successive states in canine left ventricular myocardium. II. Fiber angle and sarcomere length. Circ Res 33:656–664.
- ↑ Ross MA,Streeter DD.(1975) Nonuniform subendocardial fiber orientation in the normal macaque left ventricle. Eur J Cardiol 3:229–247
- ↑ Greenbaum RA,Yen Ho S,Gibson DG,Becker AE,Anderson RH.(1981) Left ventricular fibre architecture in man. Br Heart J 45:248–263.
- ↑ Arts T,Prinzen FW,Snoeckx LHEH,Rijcken JM,Reneman RS.(1994) Adaptation of cadiac structure by mechanical feedback in the environment of the cell: a model study. Biophys J 66:953–961
- ↑ Arts T,Veenstra PC,Reneman RS.(1979) A model of the mechanics of the left ventricle. Ann Biomed Eng 7:299–318
- ↑ Bovendeerd PHM,Arts T,Huyghe JM,van Campen DH,Reneman RS.(1992) Dependence of local left ventricular wall mechanics on myocardial fiber orientation: a model study. J Biomech 25:1129–1140.
- ↑ Chadwick RS.(1982) Mechanics of the left ventricle. Biophys J 39:279–288
- ↑ Hsu, E.W., Muzikant, A.L., Matulevicius, S.A. et al., Magnetic resonance myocardial fiber-orientation mapping with direct histological correlation, Am. J. Physiol., 274, H1627–H1634,1998
- ↑ Scollan, D.F., Holmes, A., Winslow, R. et al., Histological validation of myocardial microstructure obtained from diffusion tensor magnetic resonance imaging, Am. J. Physiol., 275, H2308–H2318,1998
- ↑ Dou, J., Tseng, W.Y., Reese, T.G. et al., Combined diffusion and strain MRI reveals structure and function of human myocardial laminar sheets in vivo, Magn. Reson. Med., 50, 107–113,2003
- ↑ David E. Sosnovik, MD,Ruopeng Wang, MS,Guangping Dai, PhD,Teresa Wang, MS, Elena Aikawa, MD, PhD,Mikhael Novikov, MD,Anthony Rosenzweig, MD,Richard J. Gilbert, MD and Van J. Wedeen, MD,Diffusion Spectrum MRI Tractography Reveals the Presence of a Complex Network of Residual Myofibers in Infarcted Myocardium,Circulation: Cardiovascular Imaging. 2009; 2: 206-212
- ↑ Barrett et al. ""Ganong's Review of Medical Physiology 24e"" (2012) Table 30-3
- ↑ Sagawa, K., Maughan, L., Suga, H. et al., Cardiac Contraction and the Pressure–Volume Relationship.Oxford University Press, 1988
- ↑ Hill, A.V. (October 1938). "The heat of shortening and dynamics constants of muscles". Proc. R. Soc. Lond. B (London: Royal Society) 126 (843): 136–195. doi:10.1098/rspb.1938.0050
- ↑ Bers, D.M., Excitation-Contraction Coupling and Cardiac Contractile Force. Kluwer
- ↑ Rüegg, J.C., Calcium in Muscle Activation: A Comparative Approach, 2nd ed. Springer-Verlag, 1988
- ↑ Tözeren, A., Continuum rheology of muscle contraction and its application to cardiac contractility,Biophys. J., 47, 303–309, 1985
- ↑ a b Hunter, P.J., McCulloch, A.D., and ter Keurs, H.E., Modelling the mechanical properties of cardiac muscle, Prog. Biophys. Mol. Biol., 69, 289–331, 1998
- ↑ Kentish, J.C., Ter Keurs, H.E.D.J., Ricciari, L. et al., Comparisons between the sarcomere length force relations of intact and skinned trabeculae from rat right ventricle, Circ. Res., 58, 755–768,1986
- ↑ "Heart Valves". Heart and Stroke Encyclopedia. American Heart Association, Inc. Retrieved 2010-08-05.
{{cite web}}
: External link in
(help)|work=
- ↑ Klabunde, RE (2009-07-02). "Pressure Gradients". Cardiovascular Physiology Concepts. Richard E. Klabunde. Retrieved 2010-08-06.
- ↑ Klabunde, RE (2007-04-05). "Cardiac Valve Disease". Cardiovascular Physiology Concepts. Richard E. Klabunde. Retrieved 2010-08-06.
- ↑ Christie, G.W. 1990. Anatomy of aortic heart valve leaflets: the influence of glutaraldehyde fixation on function. Eur. J. Cardio-Thorac. Surg. 6[Supp 1]: S25–S33.
- ↑ Thubrikar, M. 1990. The Aortic Valve. CRC Press, Boca Raton, FL.
- ↑ Westaby, S., Karp, R.B., Blackstone, E.H., and Bishop, S.P. 1984. Adult human valve dimensions and their surgical significance. Am. J. Cardiol. 53: 552–556.
- ↑ Gramiak, R. and Shah, P.M. 1970. Echocardiography of the normal and diseased aortic valve. Radiology 96: 1.
- ↑ Komoda, T., Hetzer, R., Uyama, C., Siniawski, H., Maeta, H., Rosendahl, P., and Ozaki, K. 1994. Mitral annular function assessed by 3D imaging for mitral valve surgery. J. Heart Valve Dis. 3: 483–490.
- ↑ Pai, R.G., Tanimoto, M., Jintapakorn, W., Azevedo, J., Pandian, N.G., and Shah, P.M. 1995. Volume-rendered three-dimensional dynamic anatomy of the mitral annulus using a transesophageal echocardiographic technique. J. Heart Valve Dis. 4: 623–627.
- ↑ Glasson, J.R., Komeda, M., Daughters, G.T., Niczyporuk, M.A., Bolger, A.F., Ingels, N.B., and Miller, D.C.1996. Three-dimensional regional dynamics of the normal mitral annulus during left ventricular ejection. J. Thorac. Cardiovasc. Surg. 111: 574–585
- ↑ Silverman, M.E. and Hurst, J.W. 1968. The mitral complex: interaction of the anatomy, physiology, and pathology of the mitral annulus, mitral valve leaflets, chordae tendineae and papillary muscles. Am.Heart J. 76: 399–418.
- ↑ Raganathan, N., Lam, J.H.C., Wigle, E.D., and Silver, M.D. 1970. Morphology of the human mitral valve:the valve leaflets. Circulation 41: 459–467
- ↑ Roberts, W.C. 1983. Morphologic features of the normal and abnormal mitral valve. Am. J. Cardiol. 51:1005–1028
- ↑ Barlow, J.B. 1987. Perspectives on the Mitral Valve. F.A. Davis Company, Philadelphia, PA.
- ↑ Kunzelman, K.S., Cochran, R.P., Verner, E.D., and Eberhart, R.D. 1994. Anatomic basis for mitral valve modeling. J. Heart Valve Dis. 3: 491–496.
- ↑ Kunzelman, K.S., Cochran, R.P., Murphee, S.S., Ring, W.S., Verrier, E.D., and Eberhart, R.C. 1993a. Differential collagen distribution in the mitral valve and its influence on biomechanical behaviour.J. Heart Valve Dis. 2: 236–244
- ↑ Moore, K.L., & Agur, A.M. (2007). Essential Clinical Anatomy: Third Edition. Baltimore: Lippincott Williams & Wilkins. 92. ISBN 978-0-7817-6274-8
- ↑ Moore, K.L., & Agur, A.M. (2007). Essential Clinical Anatomy: Third Edition. Baltimore: Lippincott Williams & Wilkins. 94. ISBN 978-0-7817-6274-8
- ↑ a b Wikipedia, Blood Vessel.
- ↑ The Franklin Institute Inc. "Blood – The Human Heart". Retrieved 19 March 2009.
- ↑ Gerard J. Tortora, Bryan Derrickson (2012). "The Cardiovascular System: The Blood". Principles of Anatomy & Physiology, 13th. John Wiley & Sons, Inc. pp. 729–732. ISBN 978-0470-56510-0.
{{cite book}}
: Unknown parameter|ignore-isbn-error=
ignored (|isbn=
suggested) (help)CS1 maint: uses authors parameter (link) - ↑ Liepsch, D., Thurston, G., and Lee, M. 1991. Studies of fluids simulating blood-like rheological properties and applications in models of arterial branches. Biorheology 28: 39
- ↑ Gerard J. Tortora, Bryan Derrickson (2012). "The Cardiovascular System: Blood Vessels and Hemodynamics". Principles of Anatomy & Physiology, 13th. John Wiley & Sons, Inc. p. 816. ISBN 978-0470-56510-0.
{{cite book}}
: Unknown parameter|ignore-isbn-error=
ignored (|isbn=
suggested) (help)CS1 maint: uses authors parameter (link) - ↑ Wilkinson IB, Cockcroft JR, Webb DJ (1998). "Pulse wave analysis and arterial stiffness". J. Cardiovasc. Pharmacol. 32 (Suppl 3): S33–7. PMID 9883745.
{{cite journal}}
: CS1 maint: multiple names: authors list (link) - ↑ Nichols WW (January 2005). "Clinical measurement of arterial stiffness obtained from noninvasive pressure waveforms". Am. J. Hypertens. 18 (1 Pt 2): 3S–10S. doi:10.1016/j.amjhyper.2004.10.009. PMID 15683725.
- ↑ Wilkinson IB, Fuchs SA, Jansen IM; et al. (December 1998). "Reproducibility of pulse wave velocity and augmentation index measured by pulse wave analysis". J. Hypertens. 16 (12 Pt 2): 2079–84. doi:10.1097/00004872-199816121-00033. PMID 9886900.
{{cite journal}}
: Explicit use of et al. in:|author=
(help)CS1 maint: multiple names: authors list (link) - ↑ Gosling, R.G.; Budge, M.M. (2003). "Terminology for Describing the Elastic Behavior of Arteries". Hypertension. 41 (6): 1180–1182. doi:10.1161/01.HYP.0000072271.36866.2A.
- ↑ Friedman, M.H., Brinkman, A.M., Qin, J.J. et al. 1993. Relation between coronary artery geometry and the distribution of early sudanophilic lesions. Atherosclerosis 98:193.
- ↑ Klabunde, Richard E. "Venous Return - Hemodynamics". Cardiovascular Physiology Concepts. Retrieved 8 March 2011.
- ↑ Guyton, Arthur C.; John E. (John Edward) (2006). Textbook Of Medical Physiology (11th ed.). Philadelphia: Elsevier Inc. ISBN 0-7216-0240-1.
{{cite book}}
: CS1 maint: multiple names: authors list (link)Template:Pn - ↑ Gorgeia Physiology,Indirect Measurement of Cardiac Output
- ↑ wikipedia,Arterial blood
- ↑ "Arteriovenous oxygen difference". Sports Medicine, Sports Science and Kinesiology. Net Industries and its Licensors. 2011. Retrieved 30 April 2011.
- ↑ Jansen JR. The thermodilution method for the clinical assessment of cardiac output. Intensive Care Med. 1995;21:691–7.
- ↑ Breukers RB, Jansen JR. Pulmonary artery thermodilution cardiac output vs. transpulmonary thermodilution cardiac output in two patients with intrathoracic pathology. Acta Anaesthesiol Scand. 2004;48:658–61.
- ↑ Swan HJ, Ganz W, Forrester J, Marcus H, Diamond G, Chonette D. Catheterization of the heart in man with use of a flow-directed balloon-tipped catheter. N Engl J Med. 1970;283:447–51.
- ↑ a b Yelderman M. Continuous measurement of cardiac output with the use of stochastic system identification techniques. J Clin Monit. 1990;6:322–32.
- ↑ Aranda M, Mihm FG, Garrett S, Mihm MN, Pearl RG. Continuous cardiac output catheters: delay in in vitro response time after controlled flow changes. Anesthesiology. 1998;89:1592–5.
- ↑ Tibby SM, Hatherill M, Marsh MJ, Morrison G, Anderson D, Murdoch IA. Clinical validation of cardiac output measurements using femoral artery thermodilution with direct Fick in ventilated children and infants. Intensive Care Med. 1997;23:987–91.
- ↑ Sakka SG, Bredle DL, Reinhart K, Meier-Hellmann A. Comparison between intrathoracic blood volume and cardiac filling pressures in the early phase of hemodynamic instability of patients with sepsis or septic shock. J Crit Care. 1999;14:78–83.
- ↑ Pauli C, Fakler U, Genz T, Hennig M, Lorenz HP, Hess J. Cardiac output determination in children: equivalence of the transpulmonary thermodilution method to the direct Fick principle. Intensive Care Med. 2002;28:947–52.
- ↑ Jansen JR, Schreuder JJ, Settels JJ, Kornet L, Penn OC, Mulder PG, Versprille A, Wesseling KH. Single injection thermodilution. A flow-corrected method. Anesthesiology. 1996;85:481–90.
- ↑ Jansen JR, Schreuder JJ, Punt KD, van den Berg PC, Alfieri O. Mean cardiac output by thermodilution with a single controlled injection. Crit Care Med. 2001;29:1868–73.
- ↑ Band DM, Linton RA, O'Brien TK, Jonas MM, Linton NW. The shape of indicator dilution curves used for cardiac output measurement in man. J Physiol. 1997;498:225–9
- ↑ Jonas MM, Lint RAF, O'Brein TK, Band DM, Linton NW, Kelly F, Burden TJ, Chevalier SFA, Thompson RPH, Birch NJ, Powell JJ. The pharmacokinetics of intravenous lithium chloride in patients and normal volunteers. J Trace Microprobe Techn. 2001;19:313–20.
- ↑ a b Cecconi M, Fawcett J, Grounds RM, Rhodes A. A prospective study to evaluate the accuracy of pulse power analysis to monitor cardiac output in critically ill patients. BMC Anesthesiol. 2008;8:3 Invalid
<ref>
tag; name "CecconiM" defined multiple times with different content - ↑ Frank O. Die Gründform des arterielen Pulses erste Abhandlung: mathematische Analyse. Z Biol. 1899:483–526
- ↑ Halvorsen PS, Sokolov A, Cvancarova M, Hol PK, Lundblad R, Tonnessen TI. Continuous cardiac output during off-pump coronary artery bypass surgery: pulse-contour analyses vs pulmonary artery thermodilution. Br J Anaesth. 2007;99:484–92.
- ↑ Johansson A, Chew M. Reliability of continuous pulse contour cardiac output measurement during hemodynamic instability. J Clin Monit Comput. 2007;21:237–42.
- ↑ Hamzaoui O, Monnet X, Richard C, Osman D, Chemla D, Teboul JL. Effects of changes in vascular tone on the agreement between pulse contour and transpulmonary thermodilution cardiac output measurements within an up to 6-hour calibration-free period. Crit Care Med. 2008;36:434–40.
- ↑ de Wilde RB, Breukers RB, van den Berg PC, Jansen JR. Monitoring cardiac output using the femoral and radial arterial pressure waveform. Anaesthesia. 2006;61:743–6.
- ↑ Jansen JR, Wesseling KH, Settels JJ, Schreuder JJ. Continuous cardiac output monitoring by pulse contour during cardiac surgery. Eur Heart J. 1990;11(Suppl. I):26–32.
- ↑ Romano SM, Pistolesi M. Assessment of cardiac output from systemic arterial pressure in humans. Crit Care Med. 2002;30:1834–41.
- ↑ Romano, Salvatore M.; Pistolesi, Massimo (2002). "Assessment of cardiac output from systemic arterial pressure in humans". Critical Care Medicine. 30 (8): 1834–41. doi:10.1097/00003246-200208000-00027. PMID 12163802.
- ↑ Scolletta, S.; Romano, S. M.; Biagioli, B; Capannini, G; Giomarelli, P (2005). "Pressure recording analytical method (PRAM) for measurement of cardiac output during various haemodynamic states". British Journal of Anaesthesia. 95 (2): 159–65. doi:10.1093/bja/aei154. PMID 15894561.
- ↑ Calamandrei, Marco; Mirabile, Lorenzo; Muschetta, Stefania; Gensini, Gian Franco; De Simone, Luciano; Romano, Salvatore M. (2008). "Assessment of cardiac output in children: A comparison between the pressure recording analytical method and Doppler echocardiography". Pediatric Critical Care Medicine. 9 (3): 310–2. doi:10.1097/PCC.0b013e31816c7151. PMID 18446106.
- ↑ Scolletta, S.; Gregoric, I. D; Muzzi, L.; Radovancevic, B.; Frazier, O H. (2007). "Pulse wave analysis to assess systemic blood flow during mechanical biventricular support". Perfusion. 22 (1): 63–6. doi:10.1177/0267659106074784. PMID 17633137.
- ↑ Langewouters GJ, Wesseling KH, Goedhard WJ. The static elastic properties of 45 human thoracic and 20 abdominal aortas in vitro and the parameters of a new model. J Biomech. 1984;17:425–35.
- ↑ Wesseling KH, Jansen JR, Settels JJ, Schreuder JJ. Computation of aortic flow from pressure in humans using a nonlinear, three-element model. J Appl Physiol. 1993;74:2566–73.
- ↑ Jansen JR, Schreuder JJ, Mulier JP, Smith NT, Settels JJ, Wesseling KH. A comparison of cardiac output derived from the arterial pressure wave against thermodilution in cardiac surgery patients. Br J Anaesth. 2001;87:212–22.
- ↑ Mythen, Michael Gerard; Webb, A. R. (1995). "Perioperative Plasma Volume Expansion Reduces the Incidence of Gut Mucosal Hypoperfusion During Cardiac Surgery". Archives of Surgery. 130 (4): 423–9. doi:10.1001/archsurg.1995.01430040085019. PMID 7535996.
- ↑ Sinclair, Susan; James, Sally; Singer, Mervyn (1997). "Intraoperative intravascular volume optimisation and length of hospital stay after repair of proximal femoral fracture: Randomised controlled trial". BMJ. 315 (7113): 909–12. doi:10.1136/bmj.315.7113.909. PMID 9361539.
- ↑ Conway, D. H.; Mayall, R.; Abdul-Latif, M. S.; Gilligan, S.; Tackaberry, C. (2002). "Randomised controlled trial investigating the influence of intravenous fluid titration using oesophageal Doppler monitoring during bowel surgery". Anaesthesia. 57 (9): 845–9. doi:10.1046/j.1365-2044.2002.02708.x. PMID 12190747.
- ↑ Gan, Tong J.; Soppitt, Andrew; Maroof, Mohamed; El-Moalem, Habib; Robertson, Kerri M.; Moretti, Eugene; Dwane, Peter; Glass, Peter S. A. (October 2002). "Goal-directed intraoperative fluid administration reduces length of hospital stay after major surgery". Anesthesiology. 97 (4): 820–6. PMID 12357146.
- ↑ Venn, R.; Steele, A; Richardson, P; Poloniecki, J; Grounds, M; Newman, P (2002). "Randomized controlled trial to investigate influence of the fluid challenge on duration of hospital stay and perioperative morbidity in patients with hip fracturesdagger". British Journal of Anaesthesia. 88 (1): 65–71. doi:10.1093/bja/88.1.65. PMID 11881887.
- ↑ Wakeling, H. G.; McFall, M. R.; Jenkins, C. S.; Woods, W. G.; Miles, W. F.; Barclay, G. R.; Fleming, S. C. (2005). "Intraoperative oesophageal Doppler guided fluid management shortens postoperative hospital stay after major bowel surgery". British Journal of Anaesthesia. 95 (5): 634–42. doi:10.1093/bja/aei223. PMID 16155038.
- ↑ Noblett, S. E.; Snowden, C. P.; Shenton, B. K.; Horgan, A. F. (2006). "Randomized clinical trial assessing the effect of Doppler-optimized fluid management on outcome after elective colorectal resection". British Journal of Surgery. 93 (9): 1069–76. doi:10.1002/bjs.5454. PMID 16888706.
- ↑ Pillai, Praveen; McEleavy, Irene; Gaughan, Matthew; Snowden, Christopher; Nesbitt, Ian; Durkan, Garrett; Johnson, Mark; Cosgrove, Joseph; Thorpe, Andrew (2011). "A Double-Blind Randomized Controlled Clinical Trial to Assess the Effect of Doppler Optimized Intraoperative Fluid Management on Outcome Following Radical Cystectomy". The Journal of Urology. 186 (6): 2201–6. doi:10.1016/j.juro.2011.07.093. PMID 22014804.
- ↑ http://www.nice.org.uk/mtg3Template:Full
- ↑ Lowe, Graham D.; Chamberlain, Barry M.; Philpot, Eleanor J.; Willshire, Richard J. (2010). "Oesophageal Doppler Monitor (ODM) guided individualised goal directed fluid management (iGDFM) in surgery – a technical review" (PDF). Deltex Medical Technical Review.
- ↑ Rothe, CF (Feb 1993). "Mean curculatory filling pressure: its meaning and measurement". J Appl Physiol. 74 (2): 499–509. PMID 8458763.
{{cite journal}}
: Cite has empty unknown parameters:|laydate=
,|laysummary=
, and|laysource=
(help) - ↑ a b Table 30-1 in: Trudie A Goers; Washington University School of Medicine Department of Surgery; Klingensmith, Mary E; Li Ern Chen; Sean C Glasgow (2008). The Washington manual of surgery. Philadelphia: Wolters Kluwer Health/Lippincott Williams & Wilkins. ISBN 0-7817-7447-0.
{{cite book}}
: CS1 maint: multiple names: authors list (link) - ↑ a b c d Derived from values in dyn·s/cm5
- ↑ Rose, W. and Shoukas, A.A. 1993. Two-port analysis of systemic venous and arterial impedances.Am. J. Physiol. 265 (Heart Circ. Physiol. 34): H1577
- ↑ Wikipedia,Vascular resistance