Human Physiology/The endocrine system
Homeostasis — Cells — Integumentary — Nervous — Senses — Muscular — Blood — Cardiovascular — Immune — Urinary — Respiratory — Gastrointestinal — Nutrition — Endocrine — Reproduction (male) — Reproduction (female) — Pregnancy — Genetics — Development — Answers
Introduction To The Endocrine System
[edit | edit source]The endocrine system is a control system of ductless glands that secrete hormones within specific organs. Hormones act as "messengers," and are carried by the bloodstream to different cells in the body, which interpret these messages and act on them.
It seems like a far fetched idea that a small chemical can enter the bloodstream and cause an action at a distant location in the body. Yet this occurs in our bodies every day of our lives. The ability to maintain homeostasis and respond to stimuli is largely due to hormones secreted within the body. Without hormones, you could not grow, maintain a constant temperature, produce offspring, or perform the basic actions and functions that are essential for life.
The endocrine system provides an electrochemical connection from the hypothalamus of the brain to all the organs that control the body metabolism, growth and development, and reproduction.
There are two types of hormones secreted in the endocrine system: Steroidal (or lipid based) and non-steroidal, (or protein based) hormones.
The endocrine system regulates its hormones through negative feedback, except in very specific cases like childbirth. Increases in hormone activity decrease the production of that hormone. The immune system and other factors contribute as control factors also, altogether maintaining constant levels of hormones.
Types of Glands
[edit | edit source]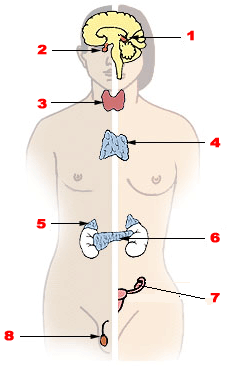
Exocrine Glands are those which release their cellular secretions through a duct which empties to the outside or into the lumen (empty internal space) of an organ. These include certain sweat glands, salivary and pancreatic glands, and mammary glands. They are not considered a part of the endocrine system.
Endocrine Glands are those glands which have no duct and release their secretions directly into the intercellular fluid or into the blood. The collection of endocrine glands makes up the endocrine system.
- 1, The main endocrine glands are the pituitary (anterior and posterior lobes), thyroid, parathyroid, adrenal (cortex and medulla), pancreas and gonads.
- 2, The pituitary gland is attached to the hypothalamus of the lower forebrain.
- 3, The thyroid gland consists of two lateral masses, connected by a cross bridge, that are attached to the trachea. They are slightly inferior to the larynx.
- 4, The parathyroid glands are four masses of tissue, two embedded posterior in each lateral mass of the thyroid gland.
- 5, One adrenal gland is located on top of each kidney. The cortex is the outer layer of the adrenal gland. The medulla is the inner core.
- 6, The pancreas is along the lower curvature of the stomach, close to where it meets the first region of the small intestine, the duodenum.
- 7, The gonads (ovaries and testes) are found in the pelvic cavity.
Hormones and Types
[edit | edit source]A hormone is a type of chemical signal. They are a means of communication between cells.
The endocrine system produces hormones that are instrumental in maintaining homeostasis and regulating reproduction and development. A hormone is a chemical messenger produced by a cell that effects specific change in the cellular activity of other cells (target cells). Unlike exocrine glands (which produce substances such as saliva, milk, stomach acid and digestive enzymes), endocrine glands do not secrete substances into ducts (tubes). Instead, endocrine glands secrete their hormones directly into the surrounding extra cellular space. The hormones then diffuse into nearby capillaries and are transported throughout the body in the blood.
The endocrine and nervous systems often work toward the same goal. Both influence other cells with chemicals (hormones and neurotransmitters). However, they attain their goals differently. Neurotransmitters act immediately (within milliseconds) on adjacent muscle, gland, or other nervous cells, and their effect is short-lived. In contrast, hormones take longer to produce their intended effect (seconds to days), may affect any cell, nearby or distant, and produce effects that last as long as they remain in the blood, which could be up to several hours.
In the following table there are the major hormones, their target and their function once in the target cell.
Endocrine Gland | Hormone Released | Chemical Class | Target Tissue/Organ | Major Function of Hormone |
---|---|---|---|---|
Hypothalamus | Hypothalamic releasing and inhibiting hormones | Peptide | Anterior pituitary | Regulate anterior pituitary hormone |
Posterior Pituitary | Antidiuretic (ADH) | Peptide | Kidneys | Stimulates water reabsorption by kidneys |
Oxytocin | Peptide | Uterus, mammary glands | Stimulates uterine muscle contractions and release of milk by mammary glands | |
Anterior Pituitary | Thyroid stimulating (TSH) | Glycoprotein | Thyroid | Stimulates thyroid |
Adrenocorticotropic (ACTH) | Peptide | Adrenal cortex | Stimulates adrenal cortex | |
Gonadotropic (FSH, LH) | Glycoprotein | Gonads | Egg and sperm production, sex hormone production | |
Prolactin (PRL) | Protein | Mammary glands | Milk production | |
Growth (GH) | Protein | Soft tissue, bones | Cell division, protein synthesis and bone growth | |
Thyroid | Thyroxine (T4) and Triiodothyronine (T3) | Iodinated amino acid | All tissue | Increase metabolic rate, regulates growth and development |
Calcitonin | Peptide | Bones, kidneys and intestine | Lowers blood calcium level | |
Parathyroids | Parathyroid (PTH) | Peptide | Bones, kidneys and intestine | Raises blood calcium level |
Adrenal Cortex | Glucocorticoids (cortisol) | Steroid | All tissue | Raise blood glucose level, stimulates breakdown of protein |
Mineralocorticoids (aldosterone) | Steroid | Kidneys | Reabsorb sodium and excrete potassium | |
Sex Hormones | Steroid | Gonads, skin, muscles and bones | Stimulates reproductive organs and brings on sex characteristics | |
Adrenal Medulla | Epinephrine and norepinephrine | Modified amino acid | Cardiac and other muscles | Released in emergency situations, raises blood glucose level, “fight or flight” response |
Pancreas | Insulin | Protein | Liver, muscles, adipose tissue | Lowers blood glucose levels, promotes formation of glycogen |
Glucagon | Protein | Liver, muscles, adipose tissue | Raises blood glucose levels | |
Testes | Androgens (testosterone) | Steroid | Gonads, skin, muscles and bone | Stimulates male sex characteristics |
Ovaries | Estrogen and progesterone | Steroid | Gonads, skin, muscles and bones | Stimulates female sex characteristics |
Thymus | Thymosins | Peptide | T lymphocytes | Stimulates production and maturation of T lymphocytes |
Pineal Gland | Melatonin | Modified amino acid | Brain | Controls circadian and circannual rhythms, possibly involved in maturation of sexual organs |
Hormones can be chemically classified into four groups:
- Amino acid-derived: Hormones that are modified amino acids.
- Polypeptide and proteins: Hormones that are chains of amino acids of less than or more than about 100 amino acids, respectively. Some protein hormones are actually glycoproteins, containing glucose or other carbohydrate groups.
- Steroids: Hormones that are lipids synthesized from cholesterol. Steroids are characterized by four interlocking carbohydrate rings.
- Eicosanoids: Are lipids synthesized from the fatty acid chains of phospholipids found in plasma membrane.
Hormones circulating in the blood diffuse into the interstitial fluids surrounding the cell. Cells with specific receptors for a hormone respond with an action that is appropriate for the cell. Because of the specificity of hormone and target cell, the effects produced by a single hormone may vary among different kinds of target cells.
Hormones activate target cells by one of two methods, depending upon the chemical nature of the hormone.
- Lipid-soluble hormones (steroid hormones and hormones of the thyroid gland) diffuse through the cell membranes of target cells. The lipid-soluble hormone then binds to a receptor protein that, in turn, activates a DNA segment that turns on specific genes. The proteins produced as result of the transcription of the genes and subsequent translation of mRNA act as enzymes that regulate specific physiological cell activity.
- Water-soluble hormones (polypeptide, protein, and most amino acid hormones) bind to a receptor protein on the plasma membrane of the cell. The receptor protein, in turn, stimulates the production of one of the following second messengers:
Cyclic AMP (cAMP) is produced when the receptor protein activates another membrane-bound protein called a G protein. The G protein activates adenylate cyclase, the enzyme that catalyzes the production of cAMP from ATP. Cyclic AMP then triggers an enzyme that generates specific cellular changes.
Inositol triphosphate (IP3) is produced from membrane phospholipids. IP3, in turn, triggers the release of CA2+ from the endoplasmic reticulum, which then activates enzymes that generate cellular changes.
Endocrine glands release hormones in response to one or more of the following stimuli:
- Hormones from other endocrine glands.
- Chemical characteristics of the blood (other than hormones).
- Neural stimulation.
Most hormone production is managed by a negative feedback system. The nervous system and certain endocrine tissues monitor various internal conditions of the body. If action is required to maintain homeostasis, hormones are released, either directly by an endocrine gland or indirectly through the action of the hypothalamus of the brain, which stimulates other endocrine glands to release hormones. The hormones activate target cells, which initiate physiological changes that adjust the body conditions. When normal conditions have been recovered, the corrective action - the production of hormones - is discontinued. Thus, in negative feedback, when the original (abnormal) condition has been repaired, or negated, corrective actions decrease or discontinue. For example, the amount of glucose in the blood controls the secretion of insulin and glucagons via negative feedback.
The production of some hormones is controlled by positive feedback. In such a system, hormones cause a condition to intensify, rather than decrease. As the condition intensifies, hormone production increases. Such positive feedback is uncommon, but does occur during childbirth, where hormone levels build with increasingly intense labor contractions. Also in lactation, hormone levels increase in response to nursing, which causes an increase in milk production. The hormone produced by the hypothalamus causing the milk let down and uterine contraction is oxytocin.
Endocrine Glands
[edit | edit source]Pituitary gland
[edit | edit source]The hypothalamus makes up the lower region of the diencephalons and lies just above the brain stem. The pituitary gland (hypophysis) is attached to the bottom of the hypothalamus by a slender stalk called the infundibulum. The pituitary gland consists of two major regions, the anterior pituitary gland (anterior lobe or adenohypophysis) and the posterior pituitary gland (posterior lobe or neurohypophysis). The hypothalamus also controls the glandular secretion of the pituitary gland.
The hypothalamus oversees many internal body conditions. It receives nervous stimuli from receptors throughout the body and monitors chemical and physical characteristics of the blood, including temperature, blood pressure, and nutrient, hormone, and water content. When deviations from homeostasis occur or when certain developmental changes are required, the hypothalamus stimulates cellular activity in various parts of the body by directing the release of hormones from the anterior and posterior pituitary glands. The hypothalamus communicates directives to these glands by one of the following two pathways: The pituitary gland is found in the inferior part of the brain and is connected by the pituitary stalk. It can be referred to as the master gland because it is the main place for everything that happens within the endocrine system. It is divided into two sections: the anterior lobe (adenohypophysis) and the posterior lobe (neurohypophysis). The Anterior pituitary is involved in sending hormones that control all other hormones of the body.
Posterior pituitary
[edit | edit source]Communication between the hypothalamus and the posterior pituitary occurs through neurosecretory cells that span the short distance between the hypothalamus and the posterior pituitary. Hormones produced by the cell bodies of the neurosecretory cells are packaged in vesicles and transported through the axon and stored in the axon terminals that lie in the posterior pituitary. When the neurosecretory cells are stimulated, the action potential generated triggers the release of the stored hormones from the axon terminals to a capillary network within the posterior pituitary. Two hormones, oxytocin and antidiuretic hormone (ADH), are produced and released this way. Decreased ADH release or decreased renal sensitivity to ADH produces a condition known as diabetes insipidus. Diabetes insipidus is characterized by polyuria (excess urine production), hypernatremia (increased blood sodium content) and polydipsia (thirst). Oxytocin is secreted by paraventricular nucleus and a small quantity is secreted by supraoptic nucleus in the hypothalamus. Oxytocin is secreted in both males and females. In females, oxytocin acts on the mammary glands and uterus. In males, oxytocin facilitates release of sperm into the urethra by causing contraction of vas deferens.
The posterior lobe is composed of neural tissue [neural ectoderm] and is derived from hypothalamus. Its function is to store oxytocin and antidiuretic hormone. When the hypothalamic neurons fire these hormones are release into the capillaries of the posterior lobe.
The posterior pituitary is, in effect, a projection of the hypothalamus. It does not produce its own hormones, but only stores and releases the hormones oxytocin and antidiuretic hormone. ADH is also known as arginine vasopressin (AVP) or simply vasopressin.
Anterior pituitary
[edit | edit source]The anterior lobe is derived from oral ectoderm and is composed of glandular epithelium. Communication between the hypothalamus and the anterior pituitary (adenohypophysis) occurs through hormones (releasing hormones and inhibiting hormones) produced by the hypothalamus and delivered to the anterior pituitary via a portal network of capillaries. It consists of three divisions: 1. pars distalis, 2. pars tuberalis, 3. pars intermedia. The releasing and inhibiting hormones are produced by specialized neurons of the hypothalamus called neurosecretory cells. The hormones are released into a capillary network or primary plexus, and transported through veins or hypophyseal portal veins, to a second capillary network or secondary plexus that supplies the anterior pituitary. The hormones then diffuse from the secondary plexus aunshine into the anterior pituitary, where they initiate the production of specific hormones by the anterior pituitary. Many of the hormones produced by the anterior pituitary are tropic hormones or tropins, which are hormones that stimulate other endocrine glands to secrete their hormones.
The anterior pituitary lobe receives releasing hormones from the hypothalamus via a portal vein system known as the hypothalamic-hypophyseal portal system.
The anterior pituitary secretes:
- thyroid-stimulating hormone (TSH)
- adrenocorticotropic hormone (ACH)
- prolactin
- follicle-stimulating hormone (FSH)
- luteinizing hormone (LH)
- growth hormone (GH)
- endorphins
- and other hormones
It does this in response to a variety of chemical signals from the hypothalamus, which travels to the anterior lobe by way of a special capillary system from the hypothalamus, down the median eminence, to the anterior lobe. These include:
- thyrotropin-releasing hormone (TRH)
- corticotropin-releasing hormone (CRH)
- dopamine (DA), also called 'prolactin inhibiting factor' (PIF)
- gonadotropin-releasing hormone (GnRH)
- growth hormone releasing hormone (GHRH)
These hormones from the hypothalamus cause release of the respective hormone from the pituitary. The control of release of hormones from the pituitary is via negative feedback from the target gland. For example homeostasis of thyroid hormones is achieved by the following mechanism; TRH from the hypothalamus stimulates the release of TSH from the anterior pituitary. The TSH, in turn, stimulates the release of thyroid hormones form the thyroid gland. The thyroid hormones then cause negative feedback, suppressing the release of TRH and TSH.
The heart, gastrointestinal tract, the placenta, the kidneys and the skin, whose major function is not the secretion of hormones, also contain some specialized cells that produce hormones.
In addition, all cells, except red blood cells secrete a class of hormones called eicosanoids. These hormones are paracrines, or local hormones, that primarily affect neighboring cells. Two groups of eicosanoids, the prostaglandins (PGs) and the leukotrienes (LTs), have a wide range of varying effects that depend upon the nature of the target cell. Eicosanoid activity, for example, may impact blood pressure, blood clotting, immune and inflammatory responses, reproductive processes, and the contraction of smooth muscles.
Antagonistic Hormones
[edit | edit source]Maintaining homeostasis often requires conditions to be limited to a narrow range. When conditions exceed the upper limit of homeostasis, specific action, usually the production of a hormone is triggered. When conditions return to normal, hormone production is discontinued. If conditions exceed the lower limits of homeostasis, a different action, usually the production of a second hormone is triggered. Hormones that act to return body conditions to within acceptable limits from opposite extremes are called antagonistic hormones. The two glands that are the most responsible for homeostasis is the thyroid and the parathyroid.
The regulation of blood glucose concentration (through negative feedback) illustrates how the endocrine system maintains homeostasis by the action of antagonistic hormones. Bundles of cells in the pancreas called the islets of Langerhans contain two kinds of cells, alpha cells and beta cells. These cells control blood glucose concentration by producing the antagonistic hormones insulin and glucagon.
Beta cells secrete insulin. When the concentration of blood glucose raises such in after eating, beta cells secret insulin into the blood. Insulin stimulates the liver and most other body cells to absorb glucose. Liver and muscle cells convert glucose to glycogen, for short term storage, and adipose cells convert glucose to fat. In response, glucose concentration decreases in the blood, and insulin secretion discontinues through negative feedback from declining levels of glucose.
Alpha cells secrete glucagon. When the concentration of blood glucose drops such as during exercise, alpha cells secrete glucagon into the blood. Glucagon stimulates the liver to release glucose. The glucose in the liver originates from the breakdown of glycogen. Glucagon also stimulates the production of ketone bodies from amino acids and fatty acids. Ketone bodies are an alternative energy source to glucose for some tissues. When blood glucose levels return to normal, glucagon secretion discontinues through negative feedback.
Another example of antagonistic hormones occurs in the maintenance of Ca2+ ion concentration in the blood. Parathyroid hormone (PTH) from the parathyroid glands increases Ca2+ in the blood by increasing Ca2+ absorption in the intestines and reabsorption in the kidneys and stimulating Ca2+ release from bones. Calcitonin (CT) produces the opposite effect by inhibiting the breakdown of bone matrix and decreasing the release of calcium in the blood.
Thyroid gland
[edit | edit source]The Thyroid gland is one of the largest endocrine glands in the body. It is positioned on the neck just below the Larynx and has two lobes with one on either side of the trachea. It is involved in the production of the hormones T3 (triiodothyronine) and T4 (thyroxine). These hormones increase the metabolic activity of the body‘s cells. The thyroid also produces and releases the hormone calcitonin (thyrocalcitonin) which contributes to the regulation of blood calcium levels. Thyrocalcitonin or calcitonin decreases the concentration of calcium in the blood. Most of the calcium removed from the blood is stored in the bones.
The thyroid hormone consists of two components, thyroxine and iodine. This hormone increases the metabolism of most body cells. A deficiency of iodine in the diet leads to the enlargement of the thyroid gland, known as a simple goiter. Hypothyroidism during early development leads to cretinism. In adults, it produces myxedema, characterized by obesity and lethargy. Hyperthyroidism leads to a condition known as exophthalmic goiter, characterized by weight loss as well as hyperactive and irritable behavior.
The thyroid gland is a two-lobed gland that manifests a remarkably powerful active transport mechanism for up-taking iodide ions from the blood. As blood flows through the gland, iodide is converted to an active form of iodine. This iodine combines with an amino acid called tyrosine. Two molecules of iodinated tyrosine then combine to form thyroxine. Following its formation, the thyroxine becomes bound to a polysaccharide-protein material called thyroglobulin. The normal thyroid gland may store several weeks supply of thyroxine in this bound form. An enzymatic splitting of the thyroxine from the thyroglobulin occurs when a specific hormone is released into the blood. This hormone, produced by the pituitary gland, is known as thyroid-stimulating hormone (TSH). TSH stimulates certain major rate-limiting steps in thyroxine secretion, and thereby alters its rate of release. A variety of bodily defects, either dietary, hereditary, or disease induced, may decrease the amount of thyroxine released into the blood. The most popular of these defects is one that results from dietary iodine deficiency. The thyroid gland enlarges, in the continued presence of TSH from the pituitary, to form a goiter. This is a futile attempt to synthesize thyroid hormones, for iodine levels that are too low. Normally, thyroid hormones act via a negative feedback loop on the pituitary to decrease stimulation of the thyroid. In goiter, the feedback loop cannot be in operation - hence continual stimulation of the thyroid and the inevitable protuberance on the neck. Formerly, the principal source of iodine came from seafood. As a result, goiter was prevalent amongst inland areas far removed from the sea. Today, the incidence of goiter has been drastically reduced by adding iodine to table salt.
Thyroxine serves to stimulate oxidative metabolism in cells; it increases the oxygen consumption and heat production of most body tissues, a notable exception being the brain. Thyroxine is also necessary for normal growth. The most likely explanation being that thyroxine promotes the effects of growth hormone on protein synthesis. The absence of thyroxine significantly reduces the ability of growth hormone to stimulate amino acid uptake and RNA synthesis. Thyroxine also plays a crucial role in the closely related area of organ development, particularly that of the central nervous system.
If there is an insufficient amount of thyroxine, a condition referred to as hypothyroidism results. Symptoms of hypothyroidism stem from the fact that there is a reduction in the rate of oxidative energy-releasing reactions within the body cells. Usually the patient shows puffy skin, sluggishness, and lowered vitality. Other symptoms of hypothyroidism include weight gain, decreased libido, inability to tolerate cold, muscle pain and spasm, and brittle nails. Hypothyroidism in children, a condition known as cretinism, can result in mental retardation, dwarfism, and permanent sexual immaturity. Sometimes the thyroid gland produces too much thyroxine, a condition known as hyperthyroidism. This condition produces symptoms such as an abnormally high body temperature, profuse sweating, high blood pressure, loss of weight, irritability, insomnia and muscular pain and weakness. It also causes the characteristic symptom of the eyeballs protruding from the skull called exophthalmia. This is surprising because it is not a symptom usually related to a fast metabolism. Hyperthyroidism has been treated by partial removal or by partial radiation destruction of the gland. More recently, several drugs that inhibit thyroid activity have been discovered, and their use is replacing the former surgical procedures. Unfortunately thyroid conditions require lifetime treatment and because of the body's need for a sensitive balance of thyroid hormone both supplementing and suppressing thyroid function can take months or even years to regulate.
T3 and T4 Function within the body
[edit | edit source]Iodine and T4 stimulate the spectacular apoptosis (programmed cell death) of the cells of the larval gills, tail and fins Transforming the aquatic, vegetarian tadpole into the terrestrial, carnivorous frog with better neurological, visuospatial, olfactory and cognitive abilities for hunting. Contrary to amphibian metamorphosis, thyroidectomy and hypothyroidism in mammals may be considered a sort of phylogenetic and metabolic regression to a former stage of reptilian life. Indeed, many disorders that seem to afflict hypothyroid humans have reptilian-like features, such as dry, hairless, scaly, cold skin and a general slowdown of metabolism, digestion, heart rate and nervous reflexes, with lethargic cerebration, hyperuricemia and hypothermia ( Venturi, 2000). ]] The Production of T3 and T4 are regulated by thyroid stimulating hormone (TSH), released by the pituitary gland, a bean shape node in the brain. TSH Production is increased when T3 and T4 levels are too low. The thyroid hormones are released throughout the body to direct the body's metabolism. They stimulate all cells within the body to work at a better metabolic rate. Without these hormones the body's cells would not be able to regulate the speed at which they performed chemical actions. Their release will be increased under certain situations such as cold temperatures when a higher metabolism is needed to generate heat. When children are born with thyroid hormone deficiency they have problems with physical growth and developmental problems. Brain development can also be severely impaired.
The significance of iodine
[edit | edit source]Thyroid hormone cannot be produced without an abundant source of iodine. The iodine concentration within the body, although significant, can be as little as 1/25th the concentration within the thyroid itself. When the thyroid is low on iodine the body will try harder to produce T3 and T4 which will often result in a swelling of the thyroid gland, resulting in a goiter.
Extrathyroidal iodine
[edit | edit source]
Iodine accounts for 65% of the molecular weight of T4 and 59% of the T3. 15–20 mg of iodine is concentrated in thyroid tissue and hormones, but 70% of the body's iodine is distributed in other tissues, including mammary glands, eyes, gastric mucosa, the cervix, and salivary glands. In the cells of these tissues iodide enters directly by sodium-iodide symporter (NIS). Its role in mammary tissue is related to fetal and neonatal development, but its role in the other tissues is unknown. It has been shown to act as an antioxidant in these tissues.
The US Food and Nutrition Board and Institute of Medicine recommended daily allowance of iodine ranges from 150 micrograms /day for adult humans to 290 micrograms /day for lactating mothers. However, the thyroid gland needs no more than 70 micrograms /day to synthesize the requisite daily amounts of T4 and T3. These higher recommended daily allowance levels of iodine seem necessary for optimal function of a number of body systems, including lactating breast, gastric mucosa, salivary glands, oral mucosa, thymus, epidermis, choroid plexus, etc.[2][3][4] Moreover, iodine can add to double bonds of docosahexaenoic acid and arachidonic acid of cellular membranes, making them less reactive to free oxygen radicals.[5]
Calcitonin
[edit | edit source]Calcitonin is a 32 amino acid polypeptide hormone. It is an additional hormone produced by the thyroid, and contributes to the regulation of blood calcium levels. Thyroid cells produce calcitonin in response to high calcium levels in the blood. This hormone will stimulate movement of calcium into the bone structure. It can also be used therapeutically for the treatment of hypercalcemia or osteoporosis. Without this hormone calcium will stay within the blood instead of moving into bones to keep them strong and growing. Its importance in humans has not been as well established as its importance in other animals.
Parathyroid gland
[edit | edit source]There are four parathyroid glands. They are small, light-colored lumps that stick out from the surface of the thyroid gland. All four glands are located on the thyroid gland. They are butterfly-shaped and located inside the neck, more specifically on both sides of the windpipe. One of the parathyroid glands most important functions is to regulate the body's calcium and phosphorus levels. Another function of the parathyroid glands is to secrete parathyroid hormone, which causes the release of the calcium present in bone to extracellular fluid. PTH does this by depressing the production of osteoblasts, special cells of the body involved in the production of bone and activating osteoclasts, other specialized cells involved in the removal of bone.
There are two major types of cells that make up parathyroid tissue:
- One of the major cells is called oxyphil cells. Their function is basically unknown.
- The second type are called chief cells. Chief cells produce parathyroid hormone.
The structure of a parathyroid gland is very different from that of a thyroid gland. The chief cells that produce parathyroid hormone are arranged in tightly-packed nests around small blood vessels, quite unlike the thyroid cells that produce thyroid hormones, which are arranged in spheres called the thyroid follicles.
PTH or Parathyroid Hormone is secreted from these four glands. It is released directly into the bloodstream and travels to its target cells which are often quite far away. It then binds to a structure called a receptor, that is found either inside or on the surface of the target cells.
Receptors bind a specific hormone and the result is a specific physiologic response, meaning a normal response of the body.
PTH finds its major target cells in bone, kidneys, and the gastrointestinal system.
Calcitonin, a hormone produced by the thyroid gland that also regulates ECF calcium levels and serves to counteract the calcium-producing effects of PTH.
The adult body contains as much as 1 kg of calcium. Most of this calcium is found in bone and teeth.
The four parathyroid glands secrete the parathyroid hormone (PTH). It opposes the effect of thyrocalcitonin. It does this by removing calcium from its storage sites in bones, releasing it into the bloodstream. It also signals the kidneys to reabsorb more of this mineral, transporting it into the blood. It also signals the small intestine to absorb more of this mineral, transporting it from the diet into the blood.
Calcium is important for steps of body metabolism. Blood cannot clot without sufficient calcium. Skeletal muscles require this mineral in order to contract. A deficiency of PTH can lead to tetany, muscle weakness due to lack of available calcium in the blood.
The parathyroid glands were long thought to be part of the thyroid or to be functionally associated with it. We now know that their close proximity to the thyroid is misleading: both developmentally and functionally, they are totally distinct from the thyroid.
The parathyroid hormone, called parathormone, regulates the calcium-phosphate balance between the blood and other tissues. Production of this hormone is directly controlled by the calcium concentration of the extracellular fluid bathing the cells of these glands. Parathormone exerts at least the following five effects: (1) it increases gastrointestinal absorption of calcium by stimulating the active transport system and moves calcium from the gut lumen into the blood; (2) it increases the movement of calcium and phosphate from bone into extracellular fluid. This is accomplished by stimulating osteoclasts to break down bone structure, thus liberating calcium phosphate into the blood. In this way, the store of calcium contained in bone is tapped; (3) it increases re-absorption of calcium by the renal tubules, thereby decreasing urinary calcium excretion; (4) it reduces the re-absorption of phosphate by the renal tubules (5)it stimulates the synthesis of 1,25-dihydrixycholecalciferol by the kidney.
The first three effects result in a higher extracellular calcium concentration. The adaptive value of the fourth is to prevent the formation of kidney stones.
If parathyroid glands are removed accidentally during surgery on the thyroid, there would be a rise in the phosphate concentration in the blood. There would also be a drop in the calcium concentration as more calcium is excreted by the kidneys and intestines, and more incorporated into the bone. This can produce serious disturbances, particularly in the muscles and nerves, which use calcium ions for normal functioning. Over activity of the parathyroid glands, which can result from a tumor on the glands, produces a weakening of the bones. This is a condition that makes them much more vulnerable to fracturing because of excessive withdrawal of calcium from the bones.
Adrenal glands
[edit | edit source]Adrenal glands are a pair of ductless glands located above the kidneys. Through hormonal secretions, the adrenal glands regulate many essential functions in the body, including biochemical balances that influence athletic training and general stress response. The glucocorticoids include corticosterone, cortisone, and hydrocortisone or cortisol. These hormones serve to stimulate the conversion of amino acids into carbohydrates which is a process known as gluconeogenesis, and the formation of glycogen by the liver. They also stimulate the formation of reserve glycogen in the tissues, such as in the muscles. The glucocorticoids also participate in lipid and protein metabolism. The cortex of the adrenal gland is known to produce over 20 hormones, but their study can be simplified by classifying them into three categories: glucocorticoids, mineralocorticoids, and sex hormones.
They are triangular-shaped glands located on top of the kidneys. They produce hormones such as estrogen, progesterone, steroids, cortisol, and cortisone, and chemicals such as adrenalin (epinephrine), norepinephrine, and dopamine. When the glands produce more or less hormones than required by the body, disease conditions may occur.
The adrenal cortex secretes at least two families of hormones, the glucocorticoids and mineral corticoids. The adrenal medulla secretes the hormones epinephrine (adrenalin) and norepinephrine (noradrenalin).
Adrenal Cortex: The hormones made by the Adrenal Cortex supply long-term responses to stress. The two major hormones produced are the Mineral Corticoids and the Glucocorticoids. The Mineral Corticoids regulate the salt and water balance, leading to the increase of blood volume and blood pressure. The Glucocorticoids are monitoring the ACTH, in turn regulating carbohydrates, proteins, and fat metabolism. This causes an increase in blood glucose. Glucocorticoids also reduce the body's inflammatory response.
Cortisol is one of the most active glucocorticoids. It usually reduces the effects of inflammation or swelling throughout the body. It also stimulates the production of glucose from fats and proteins, which is a process referred to as gluconeogenesis.
Aldosterone is one example of a mineralocorticoid. It signals the tubules in the kidney nephrons to reabsorb sodium while secreting or eliminating potassium. If sodium levels are low in the blood, the kidney secretes more renin, which is an enzyme that stimulates the formation of angiotensin from a molecule made from the liver. Angiotensin stimulates aldosterone secretion. As a result, more sodium is reabsorbed as it enters the blood.
Aldosterone, the major mineralocorticoid, stimulates the cells of the distal convoluted tubules of the kidneys to decrease re-absorption of potassium and increase re-absorption of sodium. This in turn leads to an increased re-absorption of chloride and water. These hormones, together with such hormones as insulin and glucagon, are important regulators of the ionic environment of the internal fluid.
The renin-angiotensin-aldosterone mechanism can raise blood pressure if it tends to drop. It does this in two ways. Angiotensin is a vasoconstrictor, decreasing the diameter of blood vessels. As vessels constrict, blood pressure increases. In addition, as sodium is reabsorbed, the blood passing through the kidney becomes more hypertonic. Water follows the sodium into the hypertonic blood by osmosis. This increases the amount of volume in the blood and also increases the blood pressure.
Adrenal Medulla The hypothalamus starts nerve impulses that travel the path from the bloodstream, spinal cord, and sympathetic nerve fibers to the Adrenal Medulla, which then releases hormones. The effects of these hormones provide a short-term response to stress.
Excessive secretion of the glucocorticoids causes Cushing's syndrome, characterized by muscle atrophy or degeneration and hypertension or high blood pressure. Under secretion of these substances produces Addison's disease, characterized by low blood pressure and stress.
Epinephrine and norepinephrine produce the "fight or flight" response, similar to the effect from the sympathetic nervous system. Therefore, they increase heart rate, breathing rate, blood flow to most skeletal muscles, and the concentration of glucose in the blood. They decrease blood flow to the digestive organs and diminish most digestive processes.


The adrenal sex hormones consist mainly of male sex hormones (androgens) and lesser amounts of female sex hormones (estrogens and progesterone). Normally, the sex hormones released from the adrenal cortex are insignificant due to the low concentration of secretion. However, in cases of excess secretion, masculine or feminine effects appear. The most common syndrome of this sort is "virilism" of the female.
Should there be an insufficient supply of cortical hormones, a condition known as Addison's disease would result. This disease is characterized by an excessive excretion of sodium ions, and hence water, due to lack of mineralocorticoids. Accompanying this is a decreased blood glucose level due to a deficient supply of glucocorticoids. The effect of a decreased androgen supply cannot be observed immediately. Injections of adrenal cortical hormones promptly relieve these symptoms.
Hormonal production in the adrenal cortex is directly controlled by the anterior pituitary hormone called adrenocorticotropic hormone (ACTH).
The two adrenal glands lie very close to the kidneys. Each adrenal gland is actually a double gland, composed of an inner core like medulla and an outer cortex. Each of these is functionally unrelated.
The adrenal medulla secretes two hormones, adrenalin or epinephrine and noradrenalin or norepinephrine, whose functions are very similar but not identical. The adrenal medulla is derived embryologically from neural tissue. It has been likened to an overgrown sympathetic ganglion whose cell bodies do not send out nerve fibers, but release their active substances directly into the blood, thereby fulfilling the criteria for an endocrine gland. In controlling epinephrine secretion, the adrenal medulla behaves just like any sympathetic ganglion, and is dependent upon stimulation by sympathetic preganglionic fibers.
Epinephrine promotes several responses, all of which are helpful in coping with emergencies: the blood pressure rises, the heart rate increases, the glucose content of the blood rises because of glycogen breakdown, the spleen contracts and squeezes out a reserve supply of blood, the clotting time decreases, the pupils dilate, the blood flow to skeletal muscles increase, the blood supply to intestinal smooth muscle decreases and hairs become erect. These adrenal functions, which mobilize the resources of the body in emergencies, have been called the fight-or-flight response. Norepinephrine stimulates reactions similar to those produced by epinephrine, but is less effective in conversion of glycogen to glucose.
The significance of the adrenal medulla may seem questionable since the complete removal of the gland causes few noticeable changes; humans can still exhibit the flight-or-fight response. This occurs because the sympathetic nervous system complements the adrenal medulla in stimulating the fight-or-flight response, and the absence of the hormonal control will be compensated for by the nervous system.
Pancreas
[edit | edit source]The pancreas is very important organ in the digestion system and the circulatory system because it helps to maintain our blood sugar levels. The pancreas is considered to be part of the gastrointestinal system. It produces digestive enzymes to be released into the small intestine to aid in reducing food particles to basic elements that can be absorbed by the intestine and used by the body. It has another very different function in that it forms insulin, glucagon and other hormones to be sent into the bloodstream to regulate blood sugar levels and other activities throughout the body.
It has a pear-shape to it and is approximately 6 inches long. It is located in the middle and back portion of the abdomen. The pancreas is connected to the first part of the small intestine, the duodenum, and lies behind the stomach. The pancreas is made up of glandular tissue: any substance secreted by the cells of the pancreas will be secreted outside of the organ.
The digestive juices produced by the pancreas are secreted into the duodenum via a Y-shaped duct, at the point where the common bile duct from the liver and the pancreatic duct join just before entering the duodenum. The digestive enzymes carried into the duodenum are representative of the exocrine function of the pancreas, in which specific substances are made to be passed directly into another organ.
The pancreas is both an exocrine and an endocrine organ.
The pancreas is unusual among the body's glands in that it also has a very important endocrine function. Small groups of special cells called islet cells throughout the organ make the hormones of insulin and glucagon. These, of course, are hormones that are critical in regulating blood sugar levels. These hormones are secreted directly into the bloodstream to affect organs all over the body.
Insulin acts to lower blood sugar levels by allowing the sugar to flow into cells. Glucagon acts to raise blood sugar levels by causing glucose to be released into the circulation from its storage sites. Insulin and glucagon act in an opposite but balanced fashion to keep blood sugar levels stable.
A healthy working pancreas in the human body is important for maintaining good health by preventing malnutrition, and maintaining normal levels of blood sugar. The digestive tract needs the help of the enzymes produced by the pancreas to reduce food particles to their simplest elements, or the nutrients cannot be absorbed. Carbohydrates must be broken down into individual sugar molecules. Proteins must be reduced to simple amino acids. Fats must be broken down into fatty acids. The pancreatic enzymes are important in all these transformations. The basic particles can then easily be transported into the cells that line the intestine, and from there they can be further altered and transported to different tissues in the body as fuel sources and construction materials. Similarly, the body cannot maintain normal blood sugar levels without the balanced action of insulin and glucagon.
The pancreas contains exocrine and endocrine cells. Groups of endocrine cells, the islets of Langerhans, secrete two hormones. The beta cells secrete insulin; the alpha cells secrete glucagon. The level of sugar in the blood depends on the opposing action of these two hormones.
Insulin decreases the concentration of glucose in the blood. Most of the glucose enters the cells of the liver and skeletal muscles. In these cells, this monosaccharide is converted to the polysaccharide glycogen. Therefore, insulin promotes glycogenesis or glycogen synthesis, in which glucose molecules are added to chains of glycogen. Excess glucose is also stored as fat in adipose tissue cells in response to insulin.
Insulin deficiency leads to the development of diabetes mellitus, specifically type I, juvenile diabetes. As the pancreas does not produce sufficient insulin, it is treated by insulin injections. In type II or maturity onset diabetes, the pancreas does produce enough insulin, but the target cells do not respond to it.
As already stated, the pancreas is a mixed gland having both endocrine and exocrine functions. The exocrine portion secretes digestive enzymes into the duodenum via the pancreatic duct. The endocrine portion secretes two hormones, insulin and glucagon, into the blood.
Insulin is a hormone that acts directly or indirectly on most tissues of the body, with the exception of the brain. The most important action of insulin is the stimulation of the uptake of glucose by many tissues, particularly the liver, muscle and fat. The uptake of glucose by the cells decreases blood glucose and increases the availability of glucose for the cellular reactions in which glucose participates. Thus, glucose oxidation, fat synthesis, and glycogen synthesis are all accentuated by an uptake of glucose. It is important to note that insulin does not alter glucose uptake by the brain, nor does it influence the active transport of glucose across the renal tubules and gastrointestinal epithelium.
As stated, insulin stimulates glycogen synthesis. It also increases the activity of the enzyme that catalyzes the rate-limiting step in glycogen synthesis. Insulin also increases triglyceride levels by inhibiting triglyceride breakdown, and by stimulating production of triglyceride through fatty acid and glycerophosphate synthesis. The net protein synthesis is also increased by insulin, which stimulates the active membrane transport of amino acids, particularly into muscle cells. Insulin also has effects on other liver enzymes, but the precise mechanisms by which insulin induces these changes are not well understood.
Insulin is secreted by beta cells, which are located in the part of the pancreas known as the islets of Langerhans. These groups of cells, which are located randomly throughout the pancreas, also consist of other secretory cells called alpha cells. It is these alpha cells that secrete glucagon. Glucagon is a hormone that has the following major effects: it increases hepatic synthesis of glucose from pyruvate, lactate, glycerol, and amino acids (a process called gluconeogenesis, which also raises the plasma glucose level); and it increases the breakdown of adipose tissue triglyceride, thereby raising the plasma levels of fatty acids and glycerol. The glucagon secreting alpha cells in the pancreas, like the beta cells, respond to changes in the concentration of glucose in the blood flowing through the pancreas; no other nerves or hormones are involved.
It should be noted that glucagon has the opposite effects of insulin. Glucagon elevates the plasma glucose, whereas insulin stimulates its uptake and thereby reduces plasma glucose levels; glucagon elevates fatty acid concentrations, whereas insulin converts fatty acids and glycerol into triglycerides, thereby inhibiting triglyceride breakdown.
The alpha and beta cells of the pancreas make up a push-pull system for regulating the plasma glucose level.
Sex organs
[edit | edit source]The Sex organs (Gonads) are the testes in the male, and the ovaries in the female. Both of these organs produce and secrete hormones that are balanced by the hypothalamus and pituitary glands.
The main hormones from the reproductive organs are:
Testosterone is more prominent in males. It belongs to the family of androgens, which are steroid hormones producing masculine effects. Testosterone stimulates the development and functioning of the primary sex organs. It also stimulates the development and maintenance of secondary male characteristics, such as hair growth on the face and the deep pitch of the voice.
Estrogen In females, this hormone stimulates the development of the uterus and vagina. It is also responsible for the development and maintenance of secondary female characteristics, such as fat distribution throughout the body and the width of the pelvis.
Male
[edit | edit source]
The testes produce androgens (i.e., "testosterone"). Testosterone is classified as a steroid and is responsible for many of the physical characteristics in males like.
- Broad shoulders
- Muscular body
- Hair
Testosterone increases protein production. Hormones that build up protein are called anabolic steroids. Anabolic steroids are available commercially and are being used by athletes because they help improve their physical ability, however, they do have major side effects such as:
- Liver and kidney disorders
- Hypertension (high blood pressure)
- Decreased sperm count and impotency
- Aggressive behavior ("roid rage")
- Balding
- Acne
Female
[edit | edit source]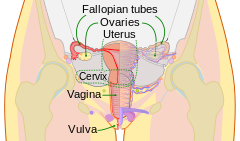
The ovaries produce estrogen and progesterone. Estrogen increases at the time of puberty and causes the growth of the uterus and vagina. Without estrogen egg maturation would not occur. Estrogen is also responsible for secondary sex characteristics such as female body hair and fat distribution. Estrogen and Progesterone are responsible for the development of the breast and for the uterine cycle. Progesterone is a female hormone secreted by the corpus luteum after ovulation during the second half of the menstrual cycle. It prepares the lining of the uterus for implantation of a fertilized egg and allows for complete shedding of the endometrium at the time of menstruation. In the event of pregnancy, the progesterone level remains stable beginning a week or so after conception.
Pineal gland
[edit | edit source]The pineal gland (also called the pineal body or epiphysis) is a small endocrine gland in the brain. It is located near the center of the brain, between the two hemispheres, tucked in a groove where the two rounded thalamic bodies join. It consists of two types of cells 1. parenchymal cells 2. neuroglial cells.
The pineal gland is a reddish-gray body about the size of a pea (8 mm in humans) located just rostro-dorsal to the superior colliculus and behind and beneath the stria medullaris, between the laterally positioned thalamic bodies. It is part of the epithalamus.
The pineal gland is a midline structure, and is often seen in plain skull X-rays, as it is often calcified. The main hormone produced and secreted by the pineal gland is melatonin. Secretion is highest at night and between the ages of 0-5. Melatonin acts mainly on gonads.
Glossary
[edit | edit source]Adrenal Gland: endocrine gland that is located on top of each kidney
Amino Acid-derived: hormones that are modified amino acids
Antagonistic Hormones: hormones that act to return body conditions to within acceptable limits from opposite extremes
Calcitonin: hormone produced by the thyroid; contributes to the regulation of blood calcium levels
Eicosanoids: lipids that are synthesized from the fatty acid chains of phospholipids found in plasma membrane
Endocrine Glands: glands that have no duct and release their secretions directly into the intercellular fluid or into the blood
Endocrine System: a control system of ductless glands that secrete chemical messengers called hormones
Estrogen: hormone in females; stimulates the development of the uterus and vagina
Exocrine Glands: glands that release their cellular secretions through a duct which empties to the outside or into the lumen (empty internal space) of an organ
Hormone: a specific chemical substance produced by certain cells that control, or help to control, cellular processes elsewhere in an organism
Insulin: hormone that acts to lower blood sugar levels by allowing the sugar to flow into cells
Iodine: chemical in the body; Thyroid hormone can not be produced with out it
Lipid-soluble Hormones: diffuse through the cell membranes of target cells
Parathyroid: four masses of tissue, two embedded posterior in each lateral mass of the thyroid gland
Pancreas: organ involved with the digestion system and the circulatory system; helps to maintain blood sugar levels
Pineal Gland: small endocrine gland in the brain located near the center of the brain, between the two hemispheres, tucked in a groove where the two rounded thalamic bodies join
Pituitary Gland: endocrine gland that is attached to the hypothalamus of the lower forebrain
Polypeptide and Proteins: hormones that are chains of amino acids of less than or more than about 100 amino acids
Steroids: hormones that are lipids that are synthesized from cholesterol; characterized by four interlocking carbohydrate rings
Testosterone: hormone more prominent in males; belongs to the family of androgens, which are steroid hormones producing masculinizing effects
Thyroid Gland: endocrine gland that consists of two lateral masses that are attached to the trachea
Thyroxine: serves to stimulate oxidative metabolism in cells; increases the oxygen consumption and heat production of most body tissues
Water-soluble Hormones: bind to a receptor protein on the plasma membrane of the cell
Chapter Review Questions
[edit | edit source]- Answers for these questions can be found here
1. My child just fell and was hurt, the anxious feeling that I feel is caused by:
- A) glucagon
- B) insulin
- C) epinephrine
- D) adrenocorticotropic
- E) None of these
2. All of Bob’s life he has had to take insulin shots, this is caused because
- A) his beta cells don’t function correctly
- B) his alpha cells don’t function correctly
- C) his DA hormone isn’t functioning correctly
- D) his GHRH hormone isn’t functioning correctly
3. The reason iodine is in salt is
- A) to prevent diabetes
- B) to prevent simple goiters
- C) to prevent Addison’s disease
- D) to prevent Cushing's syndrome
4. All hormones react to a negative feedback except
- A) progesterone
- B) estrogen
- C) prolactin
- D) oxytocin
- E) none of these
5. If I have a high blood calcium level it may be due to
- A) calcitonin
- B) parathyroid
- C) glucocorticoids
- D) glucagon
6. Hormones that are lipids that are synthesized from cholesterol
- A) protein
- B) amino acid-derived
- C) polypeptide
- D) steroids
- E) eicosanoids
7. This type of hormone must bind to a receptor protein on the plasma membrane of the cell
- A) water soluble
- B) lipid soluble
- C) steroid
- D) polypeptide
- E) a and d
- F) b and c
8. Endocrine glands release hormones in response to
- A) Hormones from other endocrine glands
- B) Chemical characteristics of the blood
- C) Neural stimulation
- D) All of the above
9. The anterior pituitary secretes
- A) oxytocin
- B) endorphins
- C) ADH
- D) TRH
10. Chief cells produce
- A) epinephrine
- B) glucagon
- C) insulin
- D) mineralocorticoids
- E) parathyroid hormone
11. Name the eight major endocrine glands.
12. Name the four major groups hormones can be chemically classified into.
References
[edit | edit source]- ↑ Venturi, S.; Donati, F.M.; Venturi, A.; Venturi, M. (2000). "Environmental Iodine Deficiency: A Challenge to the Evolution of Terrestrial Life?". Thyroid. 10 (8): 727–9. doi:10.1089/10507250050137851. PMID 11014322.
- ↑ Brown-Grant, K. (1961). "Extrathyroidal iodide concentrating mechanisms" (PDF). Physiol Rev. 41: 189.
- ↑ Spitzweg, C., Joba, W., Eisenmenger, W. and Heufelder, A.E. (1998). "Analysis of human sodium iodide symporter gene expression in extrathyroidal tissues and cloning of its complementary deoxyribonucleic acid from salivary gland, mammary gland, and gastric mucosa". J Clin Endocrinol Metab. 83: 1746. doi:10.1210/jc.83.5.1746.
{{cite journal}}
: CS1 maint: multiple names: authors list (link) - ↑ Banerjee, R.K., Bose, A.K., Chakraborty, t.K., de, S.K. and datta, A.G. (1985). "Peroxidase catalysed iodotyrosine formation in dispersed cells of mouse extrathyroidal tissues". J Endocrinol. 2: 159.
{{cite journal}}
: CS1 maint: multiple names: authors list (link) - ↑ Cocchi, M. and Venturi, S. Iodide, antioxidant function and Omega-6 and Omega-3 fatty acids: a new hypothesis of a biochemical cooperation? Progress in Nutrition, 2000, 2, 15-19