Lentis/Neuroprosthetics
Neuroprosthetics are devices that replace an impaired motor or sensory pathway by establishing a route for communication between brain and machine. The term brain–computer interface (BCI) is used when the device connects the brain to a computer rather than a mechanical device, like a prosthetic arm or leg. They are often divided into two categories: motor and sensory. A motor neuroprosthetic uses electrical signals from the brain to control devices according to the user’s intentions, while a sensory neuroprosthetic converts external stimuli into electrical signals intended for projection areas in the brain.[1] These devices are often implanted and designed to be minimally invasive, particularly in sensitive anatomical areas like the brain or brainstem. Neuroprosthetics can improve the quality of life for those with disabilities, and are currently being used in experiments to study the central nervous system (CNS).
History
[edit | edit source]
Neuroprosthetic research began in the mid-19th century, but it has recently boomed due to increases in computing power and speed.[2]
The first sensory neuroprosthetic was a cochlear implant developed by Dr. William F. House in 1957.[3] He faced stern opposition from the medical community during product development, and many felt that it would not work. Dr. House continued his efforts until he created a long-lasting prototype that he was satisfied with. The device was first implanted in a human in 1969, and years later the patient wrote a letter to Dr. House saying “I no longer live in a world of soundless movement and voiceless faces.” The Food and Drug Administration (FDA) approved his device for widespread use in 1984, and the agency’s deputy commissioner said “for the first time a device can, to a degree, replace an organ of the human senses.”
The first motor neuroprosthetic was a functional electrical stimulation (FES) device developed in 1961.[2] It was designed to solve foot drop in hemiplegic patients, which is a gait abnormality that makes it difficult to lift the front part of the foot. This condition is usually temporary but can become permanent if left untreated. At the time, their FES device was an unconventional treatment method that used electrodes to stimulate the peroneal nerve. Improvements to their design have made it possible to fit thousands of hemiplegic people with FES devices that are safe for daily use.
Neuroprosthetic research is only beginning to pick up speed, and significant strides have already been made to improve the quality of life for those with various disabilities. There are issues that remain to be solved, but with continued research it will one day be possible for an era of neurosurgery that focuses more on restorative techniques.
Current Research and Applications
[edit | edit source]Spelling Devices
[edit | edit source]Locked-in syndrome (LIS) is a condition in which a patient is completely paralyzed and unable to communicate but is aware of their surroundings. Lack of communication can negatively affect the patient's healthcare, and can cause significant grief between those affected and their loved ones.[4] Researchers have attempted to enable patients with LIS by developing neuroprosthetic spelling devices.
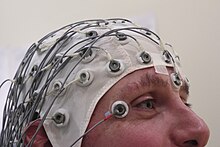
Birbaumer et al. developed a spelling device in 1999 that utilizes the slow cortical potentials (SCPs) detected by an electroencephalogram (EEG).[5] Slow cortical potentials "are shifts in the cortical electrical activity [and] might be externally triggered or self-induced".[6] These signals allowed the subjects to control the movement of a cursor depending on the type of SCP signal (positive or negative) induced. The spelling device worked by allowing patients to perform a binary search. Patients would split the alphabet into two halves, and then recursively specify the correct letter. The entire program would start again for the next letter. This method was inherently slow, and became especially cumbersome for conversation requiring the use of multiple words. Also, not all patients were successful at self-inducing SCPs.
In 2012, Sorger et al. attempted to improve the previously mentioned spelling device by utilizing functional magnetic resonance imaging (fMRI).[7] The group's spelling device associated the participant's performance of certain mental tasks to a specific blood oxygenation level-dependent (BOLD) signal. BOLD signals can be used to determine which brain region activates when the participant performs a mental task.[8] The group recorded the BOLD signals for each participant for three distinct tasks: 1) motor imagery, 2) mental calculation, and 3) inner speech. The alphabet's letters were encoded with just three BOLD signals by using three task onset delays and three task durations. For example, if the patient wanted to select the letter "E", they would wait 10 seconds from the onset of the scan and then perform a motor imagery task for 30 seconds. The fMRI-based spelling device only requires about 10 to 50 seconds in order to select a specific letter of the alphabet. However, the clinical application of fMRI-based spelling devices is limited by the cost and size of MRI machines.
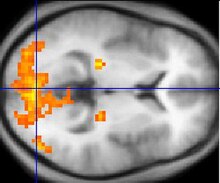
Prosthetic Limb Control
[edit | edit source]Artificial prosthetics have enabled amputee patients to perform activities, such as walking, that were once considered impossible. However, they are still crude replacements to a normal limb and are not useful for patients suffering from paralysis, such as tetraplegia. Collinger et al. developed a BCI that would enable a tetraplegic to control a prosthetic arm.[9] The group implanted two 96-channel microelectrodes into the motor cortex of an individual suffering from tetraplegia. This was done to record the individual's neural signals and develop a neural decoder machine. The neural decoder was designed to convert neural signals from the patient into digital signals that were used to control the artificial limb's movements. The participant was able to move the prosthetic limb freely in three-dimensional space and perform tasks, such as coordinated reach and grasp movements. With continued development, it is possible that BCIs can enable individuals with paralysis to use artificial limbs as naturally as an organic limb.
Neurostimulation
[edit | edit source]Neuroprosthetics are also being applied to enhance human capabilities. At HRL Laboratories, researchers are attempting to use neuroprosthetics to enhance human intellect. Using transcranial direct current stimulation (tDCS), they were able to improve the learning rate of novice subjects learning to pilot an airplane.[10] In tDCS, non-invasive electrodes are placed over the subject's head that delivers electrical currents to stimulate specific brain areas. The HRL group used EEG and Functional Near-Infrared Spectroscopy (fNIRS) to record the brain activity of expert pilots as they used a flight simulator. Next, the group used tDCS to transmit the brain activity of the expert pilots onto novice subjects during their training on the same flight simulator. The HRL group's study concluded that tDCS enhanced the piloting abilities of the novice subjects, which suggests that it may be possible to use neurostimulation as a tool to enhance human learning abilities.
Limitations and Ethics
[edit | edit source]Neuroprosthetic research has brought to light several limitations and ethical dilemmas that remain to be answered by the scientific community. First, traditional research has often relied on animal experimentation to make deductions about human anatomical and physiological phenomena. This is a limitation for neuroprosthetics because, in order to make accurate deductions, it is critical to have a test subject with a brain that is similar in complexity to the human brain. This brings up an ethical dilemma because the ideal test subject would be a human. Neuroprosthetic research is risky and can involve invasive surgical procedures in sensitive anatomical areas, which makes testing on human subjects an ethical and legal quandary. Thus, a lack of sufficient test subjects often slows progress in the field. Another limitation is that human understanding of the CNS is limited, and even procedures that are currently deemed safe can have unintended consequences in the future. For example, researchers found that deep brain stimulation, a procedure that has been used to treat a variety of neurological disorders, produced unexpected personality changes when used in patients with Parkinson's disease.[11] Finally, a major ethical dilemma involves the interplay between treatment versus enhancement. Neuroprosthetics are designed to replace a damaged motor or sensory pathway, but would it be acceptable to use these devices to augment an existing, undamaged pathway? This question has sparked great debate regarding the acceptable uses of these devices.
Controversy
[edit | edit source]Neuroprosthetic treatments are often dangerous, which puts them at a severe disadvantage when compared to alternatives. Invasive surgery carries significant risks like hemorrhage or infection. Risk assessment is made difficult by our limited understanding of the human nervous system. It seems inevitable that stimulating the brain without knowing the mechanisms at play will lead to unforeseeable consequences. As a result, there is an unavoidable element of fear that patients treated with neuroprosthetics will no longer be "who they used to be."
Surprisingly, some communities affected by neuroprosthetics have rejected the treatments intended to help them. For example, the deaf community has argued that refusing a cochlear implant is not "condemning her to a world of meaningless silence, [but] opens the child up to membership in the Deaf community, which has a rich history, language, and value system of its own."[12] These individuals believe that their condition is not a disability but a part of their identity, and to treat it as a malady is an insult.
Controversy is further supported by the depiction of neuroprosthetics in popular culture. For example, the popular science fiction TV show Doctor Who features the Cybermen as one of its main recurring villains. They are a human species who technologically enhanced their bodies until they became emotionless machines, dedicated to converting the rest of humanity to their form. Popular culture abounds with other examples of cyborgs, often portrayed in a negative light. These fears must be addressed by the research community to minimize societal anxiety.
Availability of test subjects
[edit | edit source]Due to risks associated with neuroprosthetic treatments, finding test subjects can be difficult. Regulations put in place by the FDA are often seen as a significant hindrance by researchers. In one rather extreme example, researcher Dr. Phil Kennedy, credited with developing the first successful BCI used by a locked-in patient, decided to sidestep these regulations by having a neural implant inserted into his own brain by a doctor in Belize. Unfortunately, the surgery was not without complications, and he lost the ability to speak for several days. Because his skull never closed around the implant, he had to have the device removed after getting only four weeks of good data.[13] While legally permissible, Dr. Kennedy's actions have significant ethical importance. The research community must decide how far they are willing to go to develop their technology.
References
[edit | edit source]- ↑ Leuthardt, E., Schalk, G., Moran, D., & Ojemann, J. (2006). The Emerging World of Motor Neuroprosthetics: A Neurosurgical Perspective. Neurosurgery, 59(1), 1–14. https://doi.org/10.1227/01.neu.0000243275.01470.c0
- ↑ a b Prochazka, A., Mushahwar, V. K., & McCreery, D. B. (2001). Neural prostheses. Journal of Physiology, 533, 99–109. http://www.ncbi.nlm.nih.gov/pmc/articles/PMC2278603/
- ↑ Martin, D. (2012, December 15). Dr. William F. House, Inventor of Pioneering Ear-Implant Device, Dies at 89. The New York Times. http://www.nytimes.com/2012/12/16/health/dr-william-f-house-inventor-of-cochlear-implant-dies.html
- ↑ Laureys, S., Pellas, F., Van Eeckhout, P., Ghorbel, S., Schnakers, C., Perrin, F., … Goldman, S. (2005). The locked-in syndrome : what is it like to be conscious but paralyzed and voiceless? In S. Laureys (Ed.), Progress in Brain Research (Vol. 150, pp. 495–611). Elsevier. http://www.sciencedirect.com/science/article/pii/S0079612305500347
- ↑ Birbaumer, N., Ghanayim, N., Hinterberger, T., Iversen, I., Kotchoubey, B., Kübler, A., … Flor, H. (1999). A spelling device for the paralysed. Nature, 398(6725), 297–298. https://doi.org/10.1038/18581
- ↑ Gevensleben, H., Albrecht, B., Lütcke, H., Auer, T., Dewiputri, W. I., Schweizer, R., … Rothenberger, A. (2014). Neurofeedback of slow cortical potentials: neural mechanisms and feasibility of a placebo-controlled design in healthy adults. Frontiers in Human Neuroscience, 8. https://doi.org/10.3389/fnhum.2014.00990
- ↑ Sorger, B., Reithler, J., Dahmen, B., & Goebel, R. (2012). A Real-Time fMRI-Based Spelling Device Immediately Enabling Robust Motor-Independent Communication. Current Biology, 22(14), 1333–1338. https://doi.org/10.1016/j.cub.2012.05.022
- ↑ Posse, S., Binkofski, F., Schneider, F., Gembris, D., Frings, W., Habel, U., … Eickermann, T. (2001). A new approach to measure single-event related brain activity using real-time fMRI: feasibility of sensory, motor, and higher cognitive tasks. Human Brain Mapping, 12(1), 25–41.
- ↑ Collinger, J. L., Wodlinger, B., Downey, J. E., Wang, W., Tyler-Kabara, E. C., Weber, D. J., … Schwartz, A. B. (2013). High-performance neuroprosthetic control by an individual with tetraplegia. The Lancet, 381(9866), 557–564. https://doi.org/10.1016/S0140-6736(12)61816-9
- ↑ Choe, J., Coffman, B. A., Bergstedt, D. T., Ziegler, M. D., & Phillips, M. E. (2016). Transcranial Direct Current Stimulation Modulates Neuronal Activity and Learning in Pilot Training. Frontiers in Human Neuroscience, 10. https://doi.org/10.3389/fnhum.2016.00034
- ↑ Pham, U., Solbakk, A.-K., Skogseid, I.-M., Toft, M., Pripp, A. H., Konglund, A. E., … Malt, U. F. (2015). Personality Changes after Deep Brain Stimulation in Parkinson’s Disease. Parkinson’s Disease, 2015, 7. https://www.hindawi.com/journals/pd/2015/490507/abs/
- ↑ Crouch, R. (1997). Letting the deaf be deaf: reconsidering the use of cochlear implants in prelingually deaf children. Hastings Center Report, 27(4), 14-21.
- ↑ Engber, Daniel. (2016). The neurologist who hacked his brain - and almost lost his mind. Wired. https://www.wired.com/2016/01/phil-kennedy-mind-control-computer/