Metabolomics/Applications/Nutrition/Animal Metabolomes
Back to Previous Chapter: Databases
Next chapter: Contributors
First Category: Disease Research
Go to: Plant Metabolomes
Go back to: Animal Models
Animal Metabolomics
[edit | edit source]Introduction to Animal Metabolomics
[edit | edit source]Metabolomics is a large subject that covers the chemical fingerprint or image of metabolites in a cell or tissue at a given time depending on stimulus on that tissue or cell. This snapshot of biological processes can yield an extraordinary amount of information to the genome, phenotype, and biological processes of the cell.
In animal metabolomics, rather than exploring the metabolites and processes in a human being, animals are explored. Animals explored in the following articles and websites range from domestic and agricultural to zoo and wildlife.
The website, “Bovine Functional Genomics Laboratory” is a website that explores the agricultural aspect of animal metabolomics. The website provides information about research conducted by the Bovine Functional Genomics Lab. The lab uses many biological tools to help gain a better understanding of the cellular mechanisms that can lead to economically desirable phenotypic traits in cows. The website, “Environmental Metabolomics: The Study of Disease and Toxicity in Wildlife” is a website delves more into the environmental and wildlife side of metabolomics. The website presents information about chemical risks assesments, cancer identification in species and monitoring of conditions through metabolomics. The website, “Michigan Metabolomics and Obesity Center” is a website that explores the domestic aspect of animal metabolomics. The lab explores metabolomics through the testing of domestic animals and rodents. The goal of the research center is to give clues into lipid metabolism and solve problems with human metabolism such as diabetes and obesity.
The article, “Identifying the Future Needs for Long-Term USDA Efforts in Agricultural Animal Genomics” is an article that explores the agricultural aspect of animal metabolomics. This article examines the biological mechanisms that help create economically favorable phenotypes in livestock. The article, “Direct Sampling of Organisms from the Field and Knowledge of their Phenotype: Key Recommendations for Environmental Metabolomics” is an article that explores the zoo and wildlife aspect of animal metabolomics. The focus of this article explores the links between metabolomics and phenotypes. Information about one can ultimately yield information about the other. The article, “Biomarker Discovery in Animal Health and Disease: The Application of Post-Genomic Technologies” is an article that explores the domestic aspect of animal metabolomics. This general article explores the overall implications of animal metabolomic studies with several tools. "Metabolomic Biomarkers: Their Role in the Critical Path" discusses possibilities for using biomarkers to speed up drug development and aid in diagnosing illnesses. The article, "Genetic Resources, Genome Mapping and Evolutionary Genomics of the Pig (Sus scrofa)" explores the applications of a complete genome sequence for the domesticated pig. The article illustrates sequencing techniques and possible uses in the medical, agricultural and evolutionary biology fields.
Metabolomics is an ever-growing field of biology and biochemistry that promises to yield large amounts of discoveries and innovations.
Websites
[edit | edit source]Bovine Functional Genomics Laboratory Website
[edit | edit source]
Main Focus
[edit | edit source]The purpose of this web resource is to provide information about the research being done by the Bovine Functional Genomics Lab (BFGL), which is part of the Animal and Natural Resources Institute at the Beltsville Agricultural Research Center. The BFGL was established to improve the productive efficiency of cattle by investigating the cellular mechanisms behind desirable phenotypic traits.
New Terms
[edit | edit source]abomasum:the fourth stomach compartment in ruminants [Wikipedia.org]
fluxome:metabolic pathway fluxes in the cell [Wikipedia.org]
macronutrient:nutrients that are required by an organism in relatively large quantities, such as calcium [Wikipedia.org]
microarray:a high-density arrangement of DNA probes that represent the genome of a species. It can be used to quantify the mRNA transcripts present in a cell, to compare genome sequences, or in many other applications [Snyder]
transcriptome:the set of all mRNAs in the cell [Nelson]
Nelson, DL, Cox, MM, (2004). Lehninger’s Principles of Biochemistry: W.H. Freeman.
Snyder, L, Champness, W, (2007). Molecular Genetics of Bacteria. Washington, D.C.: ASM Press.
Summary
[edit | edit source]The Bovine Functional Genomics Lab (BFGL) website describes the wide variety of research that is being done in the areas of animal health and food animal production at the BFGL lab. The lab takes an integrative approach to its research, utilizing transcriptomics, proteomics and metabolomics to gain a comprehensive understanding of the cellular mechanisms that lead to economically desirable phenotypic traits in bovine. In particular, the physiology and development of the mammary gland, the immune system and the gastrointestinal tract of the bovine is being investigated.
For each featured research project, the goals are outlined, the approach is described and the project annual reports are given. The website provides links to the publications of BFGL research and to related journals and societies. It also has a news and events section which provides information about scientific society meetings, and describes new scientific findings and prominent research awards in the field.
"Genomic and Metabolic Bases Underlying Protein and Fat Accretion in Cattle" is an example of a research project featured on the website which uses an integrative approach. This project investigates how the metabolome, the fluxome and the transcriptome in specific tissues are affected when macronutrient supplies are altered in steer. Its goal is to understand how the metabolome-fluxome-transcriptome networks are regulated to support partitioning macronutrients towards protein accretion, fat accretion or catabolism. The project uses three complementary molecular techniques: Bovine Metabolism-Related micro-array (BMET) coupled with real-time reverse transcription-polymerase chain reaction analyses, metabolomic analysis, and fluxomic analysis. To find rate-limiting elements influencing macronutrient use, the steer are fed a forage-based diet and infused per abomasum with either water, casein or starch, or fed sodium-propionate for 42 days. According to its 2008 annual report, 25 steers were prepared for the study, treated and then slaughtered. Tissue samples were collected from each steer and laboratory analyses have been initiated.
Environmental Metabolomics: The Study of Disease and Toxicity in Wildlife
[edit | edit source]http://www.actionbioscience.org/genomic/viant.html
Main Focus
[edit | edit source]The main focus of this website is usage of metabolomics in the study of disease and toxicity in wildlife

New Terms
[edit | edit source]Metabolomics: the study of all the metabolites in biological samples.
Proteomics: the stubby of the entire complement of proteins that can be produce by a biological sample.
Post-genomics Biology: biology after the availability of complete genome sequences .
Bioinformatics: applying information technology to molecular biology.
Sentinel Species: certain species that are used to monitor various characteristics of the environment.
Biomarker: a substance that is used as an indicator of biologic states.
Summary
[edit | edit source]This website is part of actionbioscience.org which touts itself as 'an educational resource of the American Institute of Biological Sciences’, that focuses entirely on metabolomics, its integration with other fields, and current applications. The page begins with an introduction to metabolomics defining it and focusing on its advantages for disease diagnosis, specifically that it measures the phenotype of the organism in question, humans or wildlife, which changes over time and in response to various stresses. It then moves, briefly, to its applications with human disease diagnosis before quickly segueing to metabolomics uses in environmental sciences, particularly identification of cancer and chemical risk assessment of various wildlife populations. Metabolomics is being used to study the effects of both chemicals and diseases on various sentinel species being used as overall indicators of the environments health and assess the effects of stressors on the environment. It can also be used as a chemical risk assessment of household and industrial chemicals effects on the environment. Traditional tests for effects of chemical toxicity monitor a limited number of responses, where as metabolomics can monitor hundreds or thousands of individual metabolites concurrently, giving a much more intricate view of the effects of a given chemical. Metabolomics with proteomics, has been used in the study of cancer in flatfish species. The page ends with the estimation of the future of environmental metabolomics, saying that currently applications are limited by technical complexities, but as technology advances people will better be able to exploit the advantages of metabolomics as a whole. With the goal of taking incredibly minute blood samples analyzing them and relating them to the health of the environment in terms of pollution and other stressors.
Michigan Metabolomics and Obesity Center
[edit | edit source]http://www.med.umich.edu/mmoc/cores/animal.htm

Main Focus
[edit | edit source]The Michigan metabolomics and obesity center performs analysis of animal as well as human metabolite analysis using many techniques.
New Terms
[edit | edit source]GC/MS: Gas chromatography/mass spectrometry
LC/MS: Liquid chromatography/mass spectrometry
MS/MS: Tandem mass spectrometry
Isotopomer analysis: usage of isotope isomers along with NMR and mass spec to quantify the metabolism of a substrate
LPA: Lysophosphatidc acid
Summary
[edit | edit source]The Michigan Metabolomics and Obesity Center operates under the University of Michigan Health System. The MMOC was founded to support epidemiological, behavioral, and clinical and health services. These services are critical to discoveries in the preclinical arena of metabolic disease. The center’s goals are to help interaction among researchers from across the university, to enhance collaborative studies and to speed translating basic science findings into clinically useful therapies for metabolic diseases including obesity, type 2 diabetes, and related metabolic disorders. The MMOC operates sever core laboratories. These facilities include Animal Phenotyping headed by Nathan Qi, Human Phenotyping headed by Jeffrey Horowitz and Metabolomics by Jaeman Byun. The laboratories for the metabolomics core allow scientists to identify, quantify and analyze biological materials in cells, organs and other biological fluids in animals (such as rodents). The laboratory uses many instruments such as mass spectrometry (in several configurations such as gas, liquid chromatography, and tandem) as well as nuclear magnetic resonance. The facility specializes in analyzing lipids. The metabolomics laboratory changes procedure rates for operations such as extraction of total lipids from biological samples, and fatty acid composition analysis. Rates are charged by sample or time. The MMOC also provides grant opportunities to scientists and studies.
Articles
[edit | edit source]Identifying the Future Needs for Long-Term USDA Efforts in Agricultural Animal Genomics
[edit | edit source]Green RD, et. al., “Identifying Future Needs for Long-Term USDA Efforts in Agricultural Animal Genomics,” International Journal of Biological Sciences, 3, 185-191, (2007).
http://www.pubmedcentral.nih.gov/articlerender.fcgi?tool=pubmed&pubmedid=17384737

Main Focus
[edit | edit source]The purpose of this article was to summarize the conclusions and recommendations made at the USDA workshop held in Washington, D.C. in September 2004 titled “Chartering the Road Map for Long Term USDA Efforts in Agricultural Animal Genomics.” This workshop was organized to promote further research into agricultural animal genomics, with an emphasis on downstream applications that will lead to animal improvements that will benefit producers, consumers and researchers.
New Terms
[edit | edit source]BAC:bacterial artificial chromosome – a plasmid cloning vector derived from the F plasmid, which can accept very large clones of DNA. It is often used in genome-sequencing project to create DNA libraries [Snyder].
cDNA:complementary DNA – DNA that has been synthesized from mRNA by way of a reverse transcriptase. It is often used to clone genes and create DNA libraries in genome-sequencing projects [Snyder].
EST:expressed sequence tag – a short sequence of cDNA, which is often used to identify gene transcripts and to determine gene sequence [Snyder].
genome annotation:assigning biological information to a genome sequence [Snyder].
in silico:performed on a computer or via a computer simulation [Wikipedia.org].
linkage maps:map of a species genome, showing positions of known genes and gene markers relative to one another, as determined by recombination frequencies [Snyder].
QTL :quantitative trait locus – sequences of DNA that are closely related to a specific, measurable phenotype [Snyder].
radiation hybrid mapping:a technique for determining the order of genetic loci along chromosomes, which involves fusing irradiated donor cells with host cells from another species. This allows fragments of DNA from the irradiated cells to become integrated into the chromosome of the host cells. Molecular probing of the DNA from the fused cells can then be used to determine whether two or more genetic loci are located within the same fragment of donor DNA [Snyder].
RNAi:RNA interference – a cellular mechanism which controls gene expression through the action of two types of RNA molecules – microRNA and small interfering RNA – which can bind to mRNA to promote or hinder its translation [Snyder].
SNP:single nucleotide polymorphism – a variation of a single nucleotide in a DNA sequence that occurs between members of the same species [Snyder].
Snyder, L, Champness, W, (2007). Molecular Genetics of Bacteria. Washington, D.C.: ASM Press.
Summary
[edit | edit source]Molecular biology has positively affected agricultural animal research, allowing for the development of more accurate and rapid animal improvements. In September 2004, a USDA workshop was held in Washington, D.C. to determine how continued research in agricultural animal genomics should be organized, funded and directed. An emphasis was placed on the downstream applications of animal genomics research, including applications in metabolomics. The ultimate goal of this research is to understand the biological mechanisms behind economically favorable phenotypes in livestock, using an integrated biological systems approach.
The first session of the workshop addressed the priorities for structural genomics in animal genomics research. The linkage and comparative maps, SNP maps and fine QTL maps (see figure 1) being created through animal genomics research projects will allow researchers to evaluate the genetic diversity of each animal species and to develop DNA-based animal identification systems. A general consensus was reached that all genome-sequencing data should be organized into public databases to facilitate these research efforts. The workshop attendees outlined several goals for future research towards structural genomics. They recommended that: 1) the swine genome be sequenced next, 2) BAC maps and 2-fold sequence coverage be obtained, 3) cDNA libraries be developed to allow functional annotation and complete integration of genetic linkage, radiation hybrid and physical maps for horse, turkey, goat, catfish , salmon and trout genomes, 4) SNP markers be discovered and validated and haplotype maps be developed for all species to allow for fine mapping of QTL, 5) standardized population and phenotype resources for each species be developed.
The second session of the workshop focused on the priorities for functional genomics in animal genomics research. Emphasis was placed on support for downstream applications in the areas of proteomics, metabolomics and metagenomics. Research in these areas will fully capitalize on the structural genomics infrastructure and will increase knowledge of the biology underlying economically desirable phenotypes in livestock. Metabolomics will allow for the genomic characterization of systems of proteins, leading to applications in animal health and nutrition. The attendees recommended that: 1) downstream research be made a high priority, 2) standardized methods for defining phenotypes be developed, 3) bioinformatics capacity be enhanced to handle the increased complexity and volume of the resulting data, 4) an integrative systems biology perspective be taken, and 5) a long-term strategic plan for USDA animal genomics research be developed and integrated.
The third and last session of the workshop addressed the priorities for bioinformatics in animal genomics research. The databases and bioinformatics tools required to manage the vast amounts of data being accrued in this research is already available. However, these systems are spread out among many agencies and there is a widespread lack of the specially trained personnel required to use them. The attendees recommended that 1) the resources available at the various agencies be integrated, 2) special programs be developed to train researchers to use these tools, 3) standard descriptions of phenotypes be developed, and 4) USDA resources be dedicated to develop new bioinformatics tools specific to agricultural animal research.
The full benefits of the research being conducted in agricultural animal genomics will be reaped in its downstream applications in structural and functional genomics. An integrated approach which includes proteomics, metabolomics and metagenomics will lead to an understanding of how genome sequences relate to economically important phenotypes in livestock, such as phenotypes involving disease resistance, behavior, growth, product quality and reproductive ability. The USDA workshop has provided recommendations for the organization and direction of further research in agricultural animal genomics that emphasize such an integrated biological systems approach.
Direct Sampling of Organisms from the Field and Knowledge of their Phenotype: Key Recommendations for Environmental Metabolomics
[edit | edit source]Hines, A.; Oladiran, G. S.; Bignell, J. P.; Stentiford, G. D.; Viant, M. R. Direct Sampling of Organisms from the Field and Knowledge of their Phenotype: Key Recommendations for Environmental Metabolomics. Environ. Sci. Technol., 2007, 41 (9), 3375-381. DOI: 10.1021/es062745w
http://pubs.acs.org/doi/full/10.1021/es062745w?prevSearch=wildlife+metabolomics&searchHistoryKey=
Main Focus
[edit | edit source]This article main focus is evaluating metabolomics for studying wildlife. Exploration of metabolomics can help yield information about phenotypes and vice versa
New Terms
[edit | edit source]Phenotypic anchoring: the process of determining the relationship between a particular expression profile and the phenotype of an organism.
Stress-induced phenotype: a phenotype induced by some stress on the organism.
Metabolic fingerprint: characteristic metabolites found in tissues or organisms.
Laboratory Stabilization: exposure of organisms to standard conditions for a period of time before measurements .
H NMR Spectroscopy: the absorption of electromagnetic radiation to determine structural features of a compound with respect to hydrogen atoms.
Summary
[edit | edit source]The authors sought to determine the potential of metabolomics in the study of free-living wildlife. Namely could it be used to identify stress-induced phenotypes on animals collected in a natural variable environment, or did it need a period of stabilization in a controlled laboratory preceding the evaluation of the samples. They also wanted to determine how much information was needed to accurately interpret the data collected, gender and age of the organism were two examples given. Marine mussels were chosen as the organism to be evaluated and hypoxia was chosen as the stressor. Two groups of mussels were collected; half of one group was resubmerged in sea water for two hours, while the other half was exposed to air for two hours, the tissues were then harvested and preserved for later analysis; the second group was transported to a laboratory for a sixty hour stabilization period before induction of hypoxia and later harvesting. H NMR spectroscopy was used to analyze the tissues, and the resulting data was subject to stringent multivariate statistical analysis. Interestingly laboratory stabilization increased the metabolic variation of the samples, thereby masking the response to the hypoxia stressor, one possible cause posited by the authors was that the transport to the laboratory caused added stress on the mussels, altering the metabolites in the isolated tissues. Most metabolic diversity was found to be caused by gender; this stresses the importance of phenotypic anchoring in evaluation of the data. The authors concluded direct field sampling is recommended for environmental metabolomics studies as it diminishes metabolic variability and allows stress-induced changes to be more easily observed.
Biomarker Discovery in Animal Health and Disease: The Application of Post-Genomic Technologies
[edit | edit source]Rowan E. Moore, Jennifer Kirwan Biomarker Discovery in Animal Health and Disease: The Application of Post-Genomic Technologies Biomarkers Insights 2: 185-196, 2007
http://www.la-press.com/biomarker-discovery-in-animal-health-and-disease-the-application-of-po-a292

Main Focus
[edit | edit source]This article talks about the role of biomarkers in the field of animal health. These markers are used to help identify the many molecules in a tissue of biological fluid. Biomarker discovery also includes transcriptomics, proteomics and metabolomics.
New Terms
[edit | edit source]RIA: Radioimmunoassay.
EST: Expressed sequence tags.
SAGE: Serial analysis of gene expression.
MIAME: Minimum information about a microarray experiment.
Johnes’ Disease: Chronic bacterial infection of ruminants.
Summary
[edit | edit source]Animals aren't only used for food but also for companionship and labor, the result of a rapid growth in human population is a large increase in required numbers and types of animals, this is why humans are increasingly concerned about the characterization and management of their diseases and mostly why animals diseases are being studied. The cause of these diseases in animals is a very complex matter however with the usage of Biomarkers, links to clinical manifestations and outcome of a particular disease can be made. With emerging technologies such as genomics, proteomics, transcriptnomics and metabolomics, usage of small molecules in tissues can be used as markers. The first important step, identifying these biomarkers is followed by validation and finally clinical use. The ultimate goal of these markers is to provide diagnostic tests to ultimately foster better animals and to detect animal pathogens. Metabolomics in animals is a very good tool in exploring biomarkers. Biological system provides a specific response to genetic and environmental influences. As a result, exploration of the metabolites of these systems can yield sensitive biomarkers. Techniques for exploring these metabolites in tissues use technologies such as mass spectrometry (gas/liquid chromatography, tandem) as well as nuclear magnetic resonance. The biggest bottleneck for research in this field today is data analysis. The large quantity of data across many labs yields complex data sets that require extensive databases. In the post genomics world, there yields a great potential in the exploration of post genomic technologies. These technologies will pave the way to improving animal health and welfare.
Metabolomic Biomarkers: Their Role in the Critical Path
[edit | edit source]Schnackenberg, Laura K. and Richard D. Beger. Metabolomic Biomarkers: Their Role in the Critical Path. Drug Discovery Today: Technologies. 4:1, 2007
http://www.fda.gov/oc/initiatives/criticalpath/metabolomic_biomarkers.html

Main Focus
[edit | edit source]Some metabolic molecules can accurately identify an individual’s health status. Metabolic profiling analyses can be implemented to identify biomarkers for diagnosis and prognosis of illnesses and to determine drug safety and effectiveness. This method could aid in the move towards personalized medicine and the FDA’s Critical Path.
New Terms
[edit | edit source]Critical Path: the FDA’s plan to personalize medicine.
Biomarkers: a molecule that can indicate a health state.
Metabolic Profiling: a spectrum of tests that determines the degree of metabolic function.
Transcriptomics: study of gene expression at the RNA level.
Systems Biology: study of the complex interactions within biology systems.
Nuclear Magnetic Resonance Spectroscopy (NMR): a technique that uses the magnetic properties of specific nuclei to determine the chemical composition of a compound.
Summary
[edit | edit source]Metabolic profiling analyses are most often performed using high-resolution NMR and hyphenated mass spectrometry. Although both techniques are not without their disadvantages, they are more readily used as opposed to other methods because NMR is easy to replicate and hyphenated mass spectrometry is highly sensitive. The best profiling test would include both NMR and mass spectrometry.
Metabolic biomarkers can be used to identify toxic drugs during the preclinical and clinical stages of pharmaceutical development. Approximately 1 billion dollars are spent to produce a single new drug and identifying toxic or ineffective drugs earlier in the developmental stages will lower that cost along with reducing the time required for the new drug to reach the market. Merck used metabolomics in a preclinical study on a drug that is known to cause hepatotoxicity and was able to pinpoint the exact mechanism that caused the toxicity. They found that those taking the drug had a depletion of tricarboxylic acid cycle intermediates and a presence of medium-chain carboxylic acids meaning the drug interfered with fatty acid metabolism.
Metabolic profile analyses are traditionally preformed on biofluids and the most popular fluid for toxicity studies is urine. Urine is easily obtained from the subject and provides toxicity details on the entire system. It is also non-invasive so it can be used in clinical studies. Since urine can also be collected over time, toxicity can be monitored and the response and recovery period can be determined. This would mean that the same animal could be used multiple times and this would save on cost for toxicological studies by decreasing the amount of animals needed to collect enough data.
Scientists already have a foothold in using biomarkers to determine disease states. For instance, NMR and mass spectrometric methods have been used for many years to detect inborn errors of metabolism. With inborn errors of metabolism, an alteration in the DNA sequence, due to nucleotide insertion, deletion, or replacement, causes a change in enzymatic activity which interferes with the conversion of a metabolic intermediate. The concentration of this intermediate can then increase and later, released into the urine and other biofluids in an attempt to keep up homeostatic concentrations. The inborn error can be determined depending on which metabolite is detected at an increased concentration and genetics can explain the alteration in the metabolic pathway.
Metabolic profiling has also been implemented to determine the success of organ transplants. Indicators of failed kidney transplants include altered levels of biomarkers such as trimethylamine-N-oxide (TMAO), lactate, acetate, and alanine. Profiling has also been used to provide a prognosis and to diagnose illnesses such as diabetes, cirrhosis, and cancer. The ability of metabolomics to provide diagnostic information for the individual makes it a great tool in the future of personalized health care and will also allow it to play a vital role in the FDA’s Critical Path which is their effort to modernize health care and drug products.
Genetic Resources, Genome Mapping and Evolutionary Genomics of the Pig (Sus scrofa)
[edit | edit source]Chen, Kefei, Tara Baxter, William M. Muir, Martien A. Groenen, Lawrence B. Schook. Genetic Resources, Genome Mapping and Evolutionary Genomics of the Pig (Sus scrofa). International Journal of Biological Sciences. 3:153-165, 2007
http://www.pubmedcentral.nih.gov/picrender.fcgi?artid=1802013&blobtype=pdf
Main Focus
[edit | edit source]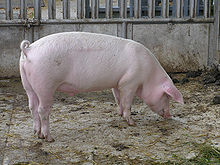
The domesticated pig is vital source of food and an important animal model for biomedical research. A complete sequence of the pig’s genome and an understanding of the intricate genetic differences between domesticated breeds will allow for improvements in pig farming and insights into human genetics. It will also give an opportunity to trace mammalian evolutionary history and define signatures of choices resulting from domestication and natural selection. This article provides a description of genome sequencing techniques and possible ways to utilize this genetic information.
New Terms
[edit | edit source]Omics: the study of biological fields ending with “omics”, such as genomics or proteomics.
Introgression gene flow from one species to another by making a hybrid of the two and then backcrossing it with one of its parents.
Germplasm: a collection of genetic resources for an organism.
Single Nucleotide Polymorphism (SNP): when a single nucleotide in an organism's DNA differs from the rest of the species.
Cytogenetics Map: a diagram depicting the location of genes are on a chromosome.
Genetic-linkage map: the distance between genes on a chromosome.
Genomic Architecture: the structure of a genome.
Summary
[edit | edit source]Developing our understanding of pig evolution could aid in determining the rise of early civilization and the origins of livestock agriculture. The earliest case of pig domestication has dated back to 7,000 BC in Antolia and since then domestication has independently arisen westward towards Europe and in the east towards Asia. Domestic pigs are found all over the globe in a variety of environments. More than 16 subspecies can be determined due to visible physical differences, such as teeth and skull morphology, along with biochemical and molecular discrepancies.
Analyzing the size and variance of diversity will give insight into the demographic history of domestication. Domestication and the novel environment farmers have created act as an artificial genetic bottleneck reducing genetic diversity and heavily selecting for the specific genes. The pig, as opposed to other domesticated livestock, is a prime model organism due to the many conspecifics in the wild that can act as outgroups when producing an evolutionary tree.
The relationship between genome size and organismal complexity has not yet been determined. However it seems that more complex organisms have a larger genome due to more elaborate measures required for regulating gene expression. Comparative genome mapping is a common approach to assess genome organization. The comparative methods are cytogenetics mapping and genetic-linkage mapping. Using comparative mapping to sequence genomes allows for a greater understanding of the molecular basis for phenotypic variation. It also provides insight into the evolutionary forces that select for a particular variation or mutation.
An analysis of genomic architecture can be used to understand species differences. Scientists look for genome rearrangements, transposable elements, and single nucleotide polymorphisms (SNPs). Genome rearrangements include gene inversions, translocations, and duplications. Chromosome rearrangements are unlikely to be the sole cause of speciation, however it can accentuate reproductive isolation already caused by other factors. Transposable elements were originally thought of as junk DNA but are now considered to be important factors in shaping the genome for speciation. Depending on where in the coding region SNPs occur, they can play a significant role in altering gene transcription.
A complete pig genome is a valuable resource. It will enhance our knowledge of the genome-phenome relationship. Pigs have experienced thousands of years of artificial selection with a large variety of morphological, physiological, and behavioral phenotypes now present, a complete genome of each breed may give a better understanding of what genotypes produce what phenotypes. This will aid in gene prediction as well as developing technologies for genetic modeling. Further exploration into genome-phenome research will offer novel applications for biotechnology and agriculture and food bioengineering. Pigs are preferred models for human biomedical research, as opposed to mice or rats, due to the similarities the two species share, such as size and many anatomical and physiological similarities. Pigs are also able to be cloned using nuclear transfer. Understanding of genotype will hone selectivity for livestock breeding purposes and allow better conservation programs to be implemented. Once the entire pig genome is untangled, it can be compared with other fully sequenced organisms and help pinpoint pig evolution as well as provide information on mammalian evolution.
Credits
[edit | edit source]This page was authored by Kevin King, Jillian Lund, Nick Chamberlain, and Kelli Fagan
Articles for future review as Metabolism class assignments
[edit | edit source]Metabolomics (liver and blood profiling) in a mouse model in response to fasting: A study of hepatic steatosis
[edit | edit source]Reviewer: Rachel R.
Main Focus
[edit | edit source]- The use of high-performance thin-layer chromatography and reversed phase liquid chromatography coupled to mass spectrometry to quantify and qualify the rearrangement and repartitioning of hepatic fat stores in mouse liver cells in response to fasting.
New Terms
[edit | edit source]- Hepatic steatosis
- also known as fatty liver disease (FLD), it is a reversible condition where large vacuoles of triglyceride fat accumulate in liver cells via the process of steatosis (abnormal retention of lipids within a cell). (source: http://en.wikipedia.org/wiki/Hepatic_steatosis)
- Hepatocytes
- make up 70-80% of the cytoplasmic mass of the liver. These cells are involved in protein synthesis, protein storage and transformation of carbohydrates, synthesis of cholesterol, bile salts and phospholipids, and detoxification, modification and excretion of exogenous and endogenous substances. (source: http://en.wikipedia.org/wiki/Hepatocytes)
- Phosphatidylcholine
- class of phospholipids that incorporate choline as a headgroup. They are a major part of biological membranes and can be isolated from either egg yolk or soybeans from which they are mechanically extracted or chemically extracted using hexane. (source: http://en.wikipedia.org/wiki/Phosphatidylcholine)
- Fibrosis
- the formation or development of excess fibrous connective tissue in an organ or tissue as a reparative or reactive process. (source: http://en.wikipedia.org/wiki/Fibrosis)
- Steatohepatitis
- a type of liver disease, characterized by inflammation of the liver with concurrent fat accumulation in liver. (source: http://en.wikipedia.org/wiki/Steatohepatitis)
- Ad libitum
- used in biology to refer to the "free-feeding" weight of an animal, as opposed, for example, to the weight after a restricted diet. (source: http://en.wikipedia.org/wiki/Ad_libitum#Biology)
- Biomarker
- a substance used as an indicator of a biological state. It is a characteristic that is objectively measured and evaluated as an indicator of normal biological processes, pathogenic processes, or pharmacologic responses to atherapeutic intervention. (source: http://en.wikipedia.org/wiki/Biomarker)
- Oleic acid
- mono-unsaturated omega-9 fatty acid found in various animal and vegetable sources. (source: http://en.wikipedia.org/wiki/Oleic_acid)
Summary
[edit | edit source]
- Hepatic steatosis, or fatty liver disease (FLD), is largely researched because it is closely connected to obesity and metabolic syndromes. Hepatic steatosis is caused by the deposition of triacylglycerols (TG) in the cytoplasm of the hepatocyte. This can cause inflammation of the cells, cell death and fibrosis (steatohepatitis).
In a mouse model, hepatic steatosis is a survival strategy when in a starved state for 24 hours, but in humans hepatic steatosis can lead to fattening of the liver. The main question in this area of research is the comprehension of the shift from a survival strategy to the occurrence of pathogenesis such as steatohepatitis. To understand this, it is important to discover how the concentration of lipid compounds in the liver changes after a period of starvation. High performance thin-layer chromatography (HPTLC) and reversed phase liquid chromatography coupled to mass spectrometry (LC-MS) were used to quantify and qualify the changes in lipid compounds in the liver.
Mice were used in a study to determine the changes in hepatic fat stores in the liver. Six mice were used as controls and fed ad libitum. The experimental group, six other mice, was starved for a 24 hour period. Afterwards, portions of the liver from all the mice were tested using HPTLC and LC-MS for their lipid compositions. Statistical Analyzing Software was used to quantitatively analyze the differences between the control and starvation groups.
After the 24 hour starvation period, a 13.5% decrease in body mass was observed. This was explained by the lack of food and water intake in the mice. There was also an excessive entry of fatty acids into the liver during this time. This is due to the hydrolysis of body fat stores during fasting and can start hepatic steatosis. This has also been seen in dogs and rodents when they are fasting. It most likely occurs in all mammals during periods of starvation when changing from carbohydrate metabolism to fat metabolism.
Large increases in 44:2-TG and 48:3-TG were seen in liver tissue after starvation when they are normally found in adipose tissue. This suggests that there is a transfer of lipids from adipose to liver tissue when carbohydrates concentrations are low. There was also a significant rise of hepatic free cholesterol and cholesterol esters after starvation. Cholesterol has an important role in membrane fluidity, so it is possible that starvation will have negative effects on the fluidity of the liver cell membranes. Also, after starvation, a 49:4-TG was observed in liver homogenate from the mice that was not seen in the control group. This TG with an odd number of carbon atoms can be used as a new biomarker for starvation-induced hepatic steatosis.
In conclusion, this study used HPTLC and LC-MS to show that the liver is highly dynamic and has an essential role in response to fasting and conversions of metabolites in lipid metabolism. In the liver, there is repartitioning and turnover of free fatty acids which causes a buildup of triacylglycerols in hepatocytes (hepatic steatosis).
Relevance to a Traditional Metabolism Course
[edit | edit source]- This article shows how the metabolism of fats and carbohydrates changes between fed and starved states in mammals. Under fed conditions, carbohydrates are used to generate ATP and the excess are converted into fatty acids. During starvation, many changes take place in mammals to get energy that they can not get because carbohydrate intake is low. Energy can be generated from proteins, fats and stored carbohydrates. The most readily available carbohydrate, glycogen, is used first, followed by fats and finally proteins. Also, when glucose is in low concentrations, free fatty acids are moved to the liver where they undergo beta oxidation. This produces acetyl coenzyme A, which can be used to form ketone bodies. These can be transported to other tissues such as skeletal, cardiac and brain tissue during periods of starvation.
Metabolomic and flux-balance analysis of age-related decline of hypoxia tolerance in Drosophila muscle tissue
[edit | edit source]Reviewer: Stephanie S.

Main Focus
[edit | edit source]Drosophila melanogaster was studied in order to determine the relevance of age in hypoxic conditions and its impact on metabolic flux and concentration levels of metabolites. Muscle tissue of the flies were analyzed to observe rates of recovery. The resulting products in both young and old flies, after being reintroduced to oxygen, were examined to better understand metabolic control in relation to age.
New Terms
[edit | edit source]- Reperfusion
- The restoration of blood flow to an organ or tissue. (source: http://www.medterms.com/script/main/art.asp?articlekey=5308)
- Normoxia
- Normal levels of oxygen. (source: http://www.medterms.com/script/main/art.asp?articlekey=33962)
- Haemolymph
- The circulatory fluid of certain invertebrates, like blood in arthropods and to lymph in other invertebrates. (source: http://www.thefreedictionary.com/Haemolymph)
- Kaplan-Meier Estimates
- Estimates the survival function from life-time data. (source: http://en.wikipedia.org/wiki/Kaplan%E2%80%93Meier_estimator)
- Hypoxia
- A pathological condition in which the body as a whole or a region of the body is deprived of adequate oxygen supply. (source: http://en.wikipedia.org/wiki/Hypoxia_(medical))
- NMR Spectroscopy
- Nuclear magnetic resonance spectroscopy is the name given to a technique which exploits the magnetic properties of certain nuclei. (source: http://en.wikipedia.org/wiki/NMR_spectroscopy)
- Proteomics
- Large-scale study of proteins, particularly their structures and functions. (source: http://en.wikipedia.org/wiki/Proteomics)
- Flux-Balance Analysis
- A useful technique for analysis of metabolic capabilities of cellular systems. (source: http://en.wikipedia.org/wiki/Flux_balance_analysis)
- Genomics
- The branch of genetics that studies organisms in terms of their genomes (their full DNA sequences). (source: http://wordnetweb.princeton.edu/perl/webwn?s=genomics)
- Trehalose
- A crystalline disaccharide C12H22O11 stored instead of starch in many fungi and found in the blood of many insects. (source: http://www.merriam-webster.com/medical/trehalose)
Summary
[edit | edit source]As organisms age, their metabolism slows and tolerance to hypoxic situations decline. Organisms must find a way to regulate their metabolism when no oxygen is available to provide energy to their body. With ages comes a decrease in maximum heart rate, motor activity and ATP production. So when placed in a hypoxic situation strokes and heart attacks may occur because of the decline in regulation and the high demand for energy.
To understand how hypoxia and age affects metabolism Drosophila melanogaster has been studied because of its close relation with human hypoxia metabolite regulation, and the organism's short life span. To determine how metabolic regulation declines the major carbohydrate energy source concentrations were viewed along with other metabolites, and the organisms recovery rate to baseline after hypoxia were studied. Two groups, one of three day old Drosophila, and one of 40 day old Drosophila were studied during a hypoxic situation where the flies became completely motionless and their hearts stopped beating. When reintroduced to oxygen the young flies had a faster recovery period and reached their baseline heartbeat and ATP levels quicker than the older flies.
Two different methods, NMR and enzymatic assays were used to find metabolite concentrations during and after hypoxia to determine metabolic fluxes in the Drosophila. The enzymatic assays were used to determine that glycogen was the main source used to produce glucose for energy by both groups of flies when oxygen isn't available, and that treholase levels remained constant during hypoxia. During recovery the 40 day old flies used more glycogen and treholase to produce glucose than the three day old flies did. NMR was used to observe changes in metabolite concentrations from the thorax of the Drosophila where their flight muscle tissue is located. The results showed that glucose concentrations in young flies increased during hypoxia then returned to their baseline in recovery, while in old flies the glucose concentration remained constant and after hypoxia decreased.
These results showed that at a younger age, the Drosophila can regulate how much glucose is being produced and use it more efficiently because the body can recover quicker in hypoxic situations. For the older flies, they have a harder time recovering from a lack of oxygen, so they use all their carbohydrate resources possible to generate glucose after hypoxia so the body can restore its ATP levels back to baseline for the body to consume. This difference in recovery rates is most likely due to damage to the mitochondria with age. When oxygen isn't available, calcium builds up because ion pumps are inhibited and thereby damages the mitochondria, which is responsible for several energy yielding pathways such as the Krebs cycle. When the oxygen is reintroduced to the body, the mitochondria can't keep up with the demand for ATP and works much slower to regenerate it. From the study it was determined that old flies produced high levels of acetate and have high levels of glycolysis and low levels of the Krebs cycle because of differences in acetyl-CoA conversion. Thereby, with reduced occurrences of the Krebs cycle and overuse of acetate production pathways, there is less ATP being produced which takes longer for the fly to reach baseline levels again.
Overall the results from the study showed with increasing age, metabolic regulation decreases and was proved when placed in a hypoxic environment. During hypoxia, metabolic control and metabolite fluxes remain relatively similar throughout all ages, however during recovery, older Drosophila use different metabolic pathways at slower rates and have build up of different metabolites due to decreased metabolic regulation.
Relevance to a Traditional Metabolism Course
[edit | edit source]The study of metabolic regulation with age relates to the metabolism class because in this study the older Drosophila have high glycolytic fluxes than Krebs cycle fluxes, so acetyl-CoA is converted to acetate through the fatty acyl-carnitine shuttle, instead of being sent through the Krebs cycle and down the electron transport chain to produce ATP. By having an over dependence on the acetate pathway, less ATP molecules are produced which results in the flies having a slower recovery to its baseline that a three day old fly would using the Krebs cycle. In metabolic regulation if a reactant, cofactor or enzyme is missing, alternate pathways are used to produce what needs to be made to reach an organism's ultimate goal of reaching homeostasis.
Metabonomics evaluations of age-related changes in the urinary compositions of male Sprague Dawley rats and effects of data normalization methods on statistical and quantitative analysis
[edit | edit source]Reviewer: Katrina H.
Main Focus
[edit | edit source]- Identify the main focus of the resource. Possible answers include specific organisms, database design, intergration of information, but there are many more possibilities as well.
New Terms
[edit | edit source]- New Term 1
- Definition. (source: http://)
- New Term 2
- Definition. (source: http://)
- New Term 3
- Definition. (source: http://)
- New Term 4
- Definition. (source: http://)
- New Term 5
- Definition. (source: http://)
- New Term 6
- Definition. (source: http://)
- New Term 7
- Definition. (source: http://)
- New Term 8
- Definition. (source: http://)
- New Term 9
- Definition. (source: http://)
- New Term 10
- Definition. (source: http://)
Summary
[edit | edit source]- Enter your article summary here. Please note that the punctuation is critical at the start (and sometimes at the end) of each entry. It should be 300-500 words. What are the main points of the article? What questions were they trying to answer? Did they find a clear answer? If so, what was it? If not, what did they find or what ideas are in tension in their findings?
Relevance to a Traditional Metabolism Course
[edit | edit source]- Enter a 100-150 word description of how the material in this article connects to a traditional metabolism course. Does the article relate to particular pathways (e.g., glycolysis, the citric acid cycle, steroid synthesis, etc.) or to regulatory mechanisms, energetics, location, integration of pathways? Does it talk about new analytical approaches or ideas? Does the article show connections to the human genome project (or other genome projects)?