Planet Earth/6i. Earth’s Surface Processes: Sedimentary Rocks and Depositional Environments
James Hutton the Father of Geology
[edit | edit source]
What makes Earth so dynamic compared to other planets can be found in the complex ways eroded sediment is transported across its surface. Earth is continually changing its surface, with the motion of sediments that erode from topographically high rocky terrain on the continents and carried to be deposited into the low basins and shallow ocean margins, as carried by rivers and wind. It is like blood, a circulatory system of sediment pumped from one place to another across the Earth.
James Hutton’s hands were covered in human blood, as he dissected cadavers at the University of Paris in 1749, his dissertation research delved into the human circulatory system, how blood pumped from the heart in arteries and into the lung, and then drained back through the veins through the heart to be oxygenated again. He was training to become a physician, a doctor, and had traveled to France to learn the trade from his native Scotland. Hutton was curious about everything he observed, often describing it in long almost stream-of-conscious writing that was as detailed as it was verbose. He planned to return to London, and continue his work as a physician, but utilized his scientific interests into making and processing dye. His knowledge and partnerships with friends back in England allowed him some money in the sale of dyes and chemicals that he had learned to extract from soot and plants, and after less than a year in London had decided to return to Scotland. He had never known his father, who sadly died when he was 3 years old, but his father had left him some farm land out in the country southeast of the city of Edinburgh where he had grown up. The farm land had laid fallow, and in 1751 he visited the farm land of his home, which sparked his interesting in farming and agriculture. With ambitious insight, James Hutton began the process of clearing the land, and like a scientist writing his observations in a book he intended to write entitled The Elements of Agriculture. Life on the farm was idyllic, and he recorded his fondness in studying the surface of the earth in his letters and with his friends. Curiosity held his thoughts as to what lay within each pit or layer of earth exposed in each ditch they dug, and how the rivers and streams carved sediment as they moved across the landscape like blood in arteries and veins in a human body that he studied back in Paris. Over the long period of twenty-five years, James Hutton wrote of these processes, the uplift of rocky terrains, the erosion by wind and rain, the vital sediments carried across the surface into the low lands and ocean coasts, of the endless continued cycle of sediment transport. Most notably he noted that “There is no vestige of a beginning, no prospect of an end.” His book was finally published in 1788, entitled the Theory of the Earth; or an investigation of the laws observable in the composition, dissolution and restoration of land upon the globe. James Hutton’s book was a lengthy discussion of the geological processes that operate on the Earth’s surface, and how over long periods of time, these processes lead to the deposition and lithification of sediments into rock. James Hutton’s ideas were radical for the time, a time in history when scientists viewed Earth’s history as short and cataclysmic, dominated by global disasters. To James Hutton, time is endless, so lengthy was the history of the Earth. Earth was shaped by the continued erosion, motion, and deposition of sediments, which would be uplifted into mountains to start the process over again. Hutton’s ideas gave rise to an interest in surface processes of the Earth, opening the door into new fields of geology, like geomorphology (the study of Earth’s landscapes), soil sciences, and sedimentary geology. In the United States, the National Academy of Science branded the newly coined term critical zone science in 2001, to describe the science of studying surface processes on Earth and how they affect agriculture and urban and industrial development on the landscape. One aspect of this science is the identification and study of depositional environments. A depositional environment is a region where transported sediments are deposited and accumulate on the Earth’s surface. These sediments can be later be buried, and through lithification turned into sedimentary rocks with compaction and heat.
Continental Depositional Environments of the Earth
[edit | edit source]Alluvial Fans
[edit | edit source]
Alluvial fans form in areas of high relief, commonly at the base of a mountain range, where there is an abundant supply of sediment from the close proximity of the uplifted terrain. These sediments are transported through mountain valleys by streams and rivers, but also debris flows, mudslides, and landslides (or other types of mass wasting, like rock falls). This sediment accumulates at the intersection of the high topography and low topography due to reduced gravitational energy in carrying these sediments further. Alluvial fans are highly dependent on infrequent seasonal events, such as periodic flooding during the spring months due to snow melt, and debris flows following heavy rains. As a result of these regions being close to the mountains, they are very susceptible to erosion, and are more rarely preserved in the ancient rock record, compared to other depositional environments. Alluvial fans are characterized by a lack of fossils, a lobate geography, texturally immature sedimentary rocks, very poorly sorted and angular grains, cross bedding, and radial patterns of incised fluvial channels. Deposits are typically oxidized red in color as the sediment is rarely below the ground water table.
Braided River Systems
[edit | edit source]

Braided rivers form in regions of high relief and typically have limited extent, as they wash out from glacial valleys or across uplifted plains. Braided rivers are characterized by their entangled channels and braided appearance when view from above. This braided pattern is due to the high load of transported sediments, and sporadic flow of water down the river. During periods of high discharge from the river, sediment is carried and becomes choked around sand bars and channels, which are repeatedly breached and become transitory. Braided river systems are likely the primordial pattern of river flow early in Earth’s history, when the surface of Earth lacked plants. The lack of roots and vegetation results in sediment filled river systems, which exhibit a braided appearance with many point bars and sand bars entangling the most direct route for the flowing water to follow. Braided river systems are highly seasonal, often located in temperate or cold regions of the Earth with little vegetation. Braided river systems are common in deserts and high plateaus, and often can be found in proximity to mountains and alluvial fans. Braided river systems are characterized by a dominance in gravel and sand size clasts, trough, planar or cross-bedding in sandstones, with little vertical succession of grain-size or shifts in the rock, with minor fining-upward trends in each bed. Fossils are rarely preserved in these environments. Flume studies, where tilted tanks filled with sand are washed by a spigot, which serves as a single source of water and allowed to flow across the sand, will mimic a braided river system in nature due to the physics of the water flow and its interaction with the sand. If the sand is unconsolidated and loose, the pattern will always result in a braided pattern of flow. However, if the sand is consolidated, cemented together or bound by vegetation and roots; a different pattern results.
Meandering River Systems
[edit | edit source]


Rivers that become confined within a single major channel, characterized by cohesive banks which are difficult to erode, will result in a meandering pattern of flow. Meandering river systems are the dominate river system since the advent of terrestrial plants. They are also found in deep canyons, where the sides of the canyon are resistant to erosion. The flow of water across a resistant substrate will lead to the channelization of that material over time. Meanders become pronounced due to the cross-sectional differences in the velocity of the flowing water. Water flowing on one side will tend to flow slightly faster, pressing into the river bank, and erode the edge forming a cut-bank along the river channel, while on the opposite side the flow is slightly slower, resulting in deposition of sediment out of the river’s load of sediment, forming a point-bar. Over time, the river will curve into a meandering pattern, as it oscillates downstream into cut-banks and point-bars on opposite sides. Such meandering pattern results in the continued deposition of lag deposits that are added along the point bars, with coarse sand-size sediment. Meandering rivers will form natural-levee deposits, which will parallel either side of the river. These natural-levee deposits are formed by the deposition of sediments during periodic floods, however they can be overtopped by larger floods along the river, resulting in the deposition of fine-grained sediments that over-ride these river channels and deposit thick deposits in large regions near the river called a flood basin or floodplain deposits. Even greater floods will result in crevasse-splay deposits when the meandering river will be flooded with water to such an extent the water flows out across very large distances beyond the flood plain, bringing thick mud and clay sediments with this flowing floodwater. Meandering river systems will nearly always demonstrate a fining upward, with the coarsest and largest sediments forming the base in a series of layers, where the river lag deposits are the first to be deposited, while the upper layers will represent floodplain deposits and be dominated by mudstone and siltstone. Meandering river systems formed by the recycled sediment moving from cut-banks to point-bars, resulting in cross-bedding and cross-lamination that drifts horizontally across the flood plain. Channels can incise, and leave a winding pattern. Once filled with sand, these channel sands can be slowly lithified into sandstone, and the flow is preserved in rocks layers in the deserts and badlands of eastern Utah, as these sandstones are resistant to weathering. The direction and flow of ancient river channels can be studied from these petrified rivers, that erode like fossilized rivers in the desert, although others maybe hidden beneath vegetation. Meandering river systems, and floodplain deposits are excellent depositional environments to look for fossils, particularly animals and plants that live on land, including dinosaurs.
Eolian Desert Systems
[edit | edit source]
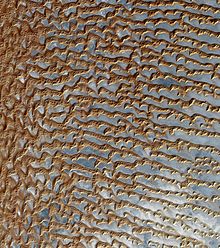

Eolian deposits can be found in regions with little vegetation due to low precipitation (rain and snow), and exposure to high winds which can carry sand. In these regions, sand and smaller grains of sediment can be transported by the blowing wind. This windblown sand can travel great distances across the surface of the Earth and even across oceans, but most often accumulate in topographical traps, where mountains or ridges rise in elevation. These topographic ridges prohibit the sand to be carried over them, and sand-size particles drop out of the air and get deposited along the edges of these ridges. Along these topographical traps, great sand dunes can form, which can be very thick. In desert regions, like in North Africa, windblown sand can cover vast regions of the continental interiors, especially in regions with little rainfall and hot temperatures. Transverse dunes are extremely long dunes of sand perpendicular to the blowing wind, and these can further lead to sand migration, as sand is continually deposited on the back side (lee side) of the dune. These thin deposits of wind-blown sand can form wide broad striations of cross beds that are much larger and sweeping than those found in fluvial depositional environments. Transverse dunes can be blown out, to form barchan sand dunes, which form a broad crescent shaped, with a steep lee side, and broad face. Sand dunes can migrate over the desert landscape, but are heavily influenced by the climate and the amount of vegetation coverage. A source of sand particles is also important, which can often come from eroding exposed sandstone. Ancient records of eolian depositional environments are found across eastern and southern Utah. The classic sandstones of the Wingate and Navajo Sandstones in Zion National Park are remains of a vast eolian desert that existed during the late Triassic and early Jurassic Periods in Utah about 200 million years ago. The artistic broadly formed cross beds of red and yellow are remains of the wind-swept sand dunes of this prehistoric desert, and these sandstones are characterized by being well sorted with orange rounded clasts of quartz dominated grains.
Lacustrine Systems
[edit | edit source]
Lacustrine systems are sedimentary depositional environments of lake systems, which act as important sources of sediment deposition. Lakes can be either be open, with the outflow of water and stable shoreline dominated by clastic sediment transport into the system, or closed with little to no outflow of water, which results in chemical sedimentation, such as carbonate sediments or if the water is subjected to evaporation and drying up – evaporitic sediments. Lakes tend to exhibit layers of laminated mud, due to the low energy to transport sediments compared to fast moving rivers. This results in thick muddy sediments that can accumulate thick deposits of clay, mud and silt on the lake floor. This mud can be cyclical, with increased sediments during periods of spring flooding, as there can be yearly increases of inflow of water and sediments. This yearly cycle can be seen within the thin layers of mud, and are called varves. Varves are thin layers of clay, mud and silt of differing color and texture which represent the deposit of a single year in a lake bottom. They can be used as a chronological history in the study of sediments laid down on a lake bottom for thousands of years. Lacustrine systems can be identified by their homogeneous layers of laminated muds that lithify into shale. The Green River Shale exposed across northeastern Utah, southwestern Wyoming and western Colorado is an example of an ancient lacustrine system representing lakes that existed at one time in the intermountain basins of this region of the United States during the Eocene Epoch, about 50 million years ago.
Deltaic Systems
[edit | edit source]
Deltas are discrete coastline protuberances formed where major rivers enter oceans, semi-enclosed seas, lakes or lagoons and supply sediment more rapidly than can be redistributed by the subsidence of the basin. Deltaic depositional environments carry sediments into basins, and these fluvially carried sand, silt, mud and clay grains accumulate along the coastline, shifting the shoreline into the ocean or sea basin, resulting in a regression of the coastline outward into the ocean. A delta is named for the triangular shape they produce as the river flows across this region in its attempt to find the most direct downslope path to the ocean, blocked by sediment deposited at this transition between land and sea. The first recognized delta is the Nile Delta near Alexander, Egypt, but other deltas include the Mississippi Delta in Louisiana, and the Ganges Delta in Bengal and Bangladesh in India. The path of the maze of tributaries of rivers across a delta are consistently changing due to sediment accumulation, and water flowing downslope through these sediments into complex channels. The result of these surface processes is a complex triangular shaped region at the intersection of a major river and the sea. They exhibit a coarsing upward in grain sizes, cross-bedding, ripple marks caused by tides, and clay and mud that flares upward through coarser overlaying grains. There is also a fair amount of bioturbation in deltas. Bioturbation is traces of organisms (like clams and crustaceans) that burrow or bore into the sediments, leaving traces of these burrows and a mix of sediments due to biological activity in the ground.
Beach and Barrier Islands
[edit | edit source]


Beaches are long, narrow accumulations of sand which are aligned parallel to a coastline near the shore, while barrier islands are accumulations of sand that are cut off from the mainland by shallow lagoons, estuaries or marshes. Beach and barrier islands are dominated by wave action along the coastline, resulting in well-polished sand grains that are continually being agitated in this high energy system. Ocean tides and wind also play a role in sediment transport along these coastal environments. Beach depositional environments can be divided into zones based on their distance and location from the shoreline which is consistently shifting with tides and crashing waves. The backshore is the region of a beach or barrier island that is above the high tide. The backshore is only inundated by the ocean during high storms, and is protected from more cyclical monthly and daily tides by a berm. A berm is a slight rise along the beach formed from the highest level of daily to weekly tides along a shore line. Berms protect the beach within the backshore, but can be eroded during storm surges, when the ocean inundates these areas of the beach. Sediment deposition in the backshore of a beach or barrier island is dominated by windblown sands, that can form sand dunes and eolian sedimentary structures. They can also be overgrown with plants and vegetation over time, and also subjected to beach erosion. Within the zone of crashing waves, where your feet would get wet walking along the beach is the foreshore. The foreshore can be divided into the swash zone and surf zone, with the portion of the beach overrun by water called the swash zone, while the surf zone is where waves crash further out from the shore. Further out into the ocean is the nearshore (also called the shoreface). This region is where the ocean water is shallow enough that it interacts with the ocean floor, resulting in breaking waves, that over extend their crest, and produce surf. This zone is defined by the region that is shallower than localized wave base. Wave base is the maximum depth at which a surface wave’s passage across the top of the ocean’s surface can cause motion deeper in the water column. When this depth interacts with sediments on the ocean floor, this results in the wave to begin to crest and break, resulting in the surf zone observed in the nearshore. The surf is a result of the dynamic interaction of ocean waves with the ocean floor, and it is within this zone that surfers can be transported into the shore riding these crashing waves. The wave base is calculated by finding half the length of the distance between wave crests. For example, as waves spaced 3 meters apart approach a beach, they will be moving the water below up to depths of 1.5 meters. When the waves reach that portion of the shoreline with depths shallower than 1.5 meters, the waves will begin to crest as they also interact with the sediments on the ocean floor, resulting in the transportation of sand size particles. Longshore currents are generated when the waves approach the shoreline at an angle, which causes sediment transport along the length of the beach or barrier island. Longshore currents can significantly move sediment up and down a shoreline, as they are carried by the motion generated by the approaching waves. Beaches and barrier islands are susceptible to erosion due to changes in sea level, tides, wind and especially storm waves, which will scour sediments and redeposit them farther seaward. Beach and barrier island sediments are characterized by being dominated by sand grain sizes, as well as those grains being very mature, well rounded and well sorted. Bivalve (clams) and brachiopod shelled organisms are common in these deposits. Ripple marks with bidirectional symmetric profiles are common, as well as planar and trough crossbedding, and bioturbation.
Estuarine and Lagoonal Systems
[edit | edit source]


Estuaries are the seaward portion of a drowned river valley system which receives sediment input from both downstream fluvial flow and upstream marine tidal sources, and are influenced by tides, waves, and fluvial processes. While lagoons are shallow stretches of water near or communicating with the sea, but separated by a low narrow strip of land. These depositional environments are especially important for marine life, as they often host brackish waters (water that is a mix of fresh and salt water). Estuary and lagoonal systems are formed during transgression events, when sea levels are high. Sediment input can be a result of fluvial and marine motion into these systems, resulting in a mix of sediment sources. This is influenced by episodic storms. Both types of regions are susceptible to evaporation, resulting in the accumulation or stratification of evaporitic deposits of salts.
Tidal Flat Systems
[edit | edit source]
Tidal flats are marshy and muddy to sandy areas that are partially uncovered by the rise and fall of tides, often protected by the action of waves by a geographic barrier. Sediment transport in tidal flats is driven by tides, rather than rivers. As the tides flow in and out of these regions, they deposit sediment into narrow tidal channels that are continually reworked by the daily to monthly flooding of these areas. Barriers to wave action are required which most often is due to salt-marsh vegetation or mangrove trees that help dampen wave action, which would result in the erosion of these tidal flats. Tidal flats are characterized by mud as the predominate sediment, with plant debris, bioturbation, and strange herringbone cross-stratification due to the in and out motion of the flowing water. Tidal flats deposits will often produce shales with burial and lithification, which can be stratified or sandwiched between evaporitic salt deposits.
Oceanic Deepwater Depositional Systems
[edit | edit source]
There exists vast regions on Earth that are beyond the shallow marine environment occupied by the continental rise, the deeper continental slope, down to the deep-sea trenches and dark abyssal ocean floor. All of these regions can accumulate sediment that is transported off of the eroding continents. The continental rise is the shallower region of the ocean that is proximal to the coastline of continents, which is surrounded by the much deeper ocean waters of the open ocean by a continental slope. As sediment is transported away from the continents and into deeper ocean waters, these sediments can over steepen the continental slope, resulting in underwater debris flows called turbidity flows. These fine-grained plumes of sediment can transport fine grain material deep and far distances along the ocean floor. These plumes of fine grain sediment can empty large amounts of tiny particles of sediment great distances on the ocean floor. The ocean floor also receives input of sediments from volcanic ash, and windblown sediment, as well as larger clasts carried out to sea by icebergs and floating ice. The largest input of sediment might be from pelagic rains of organic particles that are produced by marine organisms that live within the ocean’s water column, and sink to the ocean floor. Changes in the thermohaline circulation, acidic ocean waters and subduction can slow or stop the sediment input into these systems, which often is fairly continuous and rarely erode given their location on the ocean floor. These sediments often preserve an extraordinary complete record, with few if any gaps in deposition. As such many scientists, such as those abroad the JOIDES research vessel study sediment cores from these depositional systems to understand Earth’s past climate and oceanography.
Carbonate Depositional Systems
[edit | edit source]
Carbonate sediments are deposited primarily in shallow-marine shelf platforms, but are also present in marginal-marine environments and more rarely lacustrine systems. They form what is called a carbonate platform, such as coral reefs and atolls. Unlike the other depositional systems, carbonate deposition is a chemical and biochemical process, where organisms form their skeletons from calcium carbonate, and this is laid down as a framework of biological activity in these shallow ocean and lake waters. There can be physical processes in the rework and transport of these carbonate materials, but they are sourced from chemical and biochemical processes rather than erosion from the continents. Carbonate systems are active when there are numerous organisms that form their skeletons from calcium carbonate, but carbonate can also be deposited when ocean water becomes more basic in pH (increase in carbonate anions), and with an increase of dissolved calcium cations from continental weathering. Carbonate systems are limited to shallow waters, above the carbonate composition depth (CCD), and often most active in warm tropical ocean waters within the photic zone. This makes carbonate deposition highly affected by sea level changes, as drops in sea level can result in the chemical erosion or karstification of carbonate once exposed to more acidic rainwater. Carbonate systems are also influenced by the pH of the ocean water, as they require more basic ocean pH (8 or higher). If the ocean water becomes more acidic, carbonate will not precipitate as a solid and will dissolve. This can also cause risk to shelled marine organisms to dissolve their protective skeletons and shells. Carbonate depositional systems lead to the thick deposits of limestone that are common across much of Earth’s surface, particularly abundant in rocks that are younger than 545 million years old, when shelled multicellular organisms first appeared on Earth.
Unconformities
[edit | edit source]
Within these depositional environments you can see the daily evidence of the continued process of sediment transport and deposition across the Earth’s surface. James Hutton was profoundly interested in the nature of these processes and how they could occur for long periods of time, unyielding and ever flowing in the continued uninterrupted transport of sediment that accumulates into the observed rock layers found in Earth’s subsurface. But he was also interested in their gaps (called an unconformity), when these lithified sediments would rise up to be worn away again, continuing the cycle.
Siccar Point and the Abyss of Time
[edit | edit source]In 1788, he took a boat trip with his friends John Playfair and James Hall along the coast of Scotland searching for these gaps in the craggy rocky shores in the county of Berwickshire, when they discovered a point of rock called Siccar Point. The lower layers of rock project vertically, overlaid by horizontal rocks. The age of the rocks were unknown at the time to the early scientists, but the vast length of time for such configuration of sedimentation to occur, the uplift, the folding and re-deposition was astonishing to them. It was a window into the antiquity of the Earth. John Playfair wrote that “their minds grew giddy at looking so far back into the abyss of time.” This rock point along the Scottish coastline was the first evidence of the great length of time Earth has existed. These observations grew into the theory of uniformitarianism; that the changes on Earth’s surface and the deposition of sediments are a result the actions of a continuous and uniform process that operates even today. Later they would be summarized by the geologist Charles Lyell, as the present is the key to the past. The observed transport of sediment and deposition of these sediments has been continuous throughout Earth’s long history, and its record is preserved within the layers of lithified sediments that cover much of Earth’s continents.
Previous | Current | Next |
---|---|---|
i. Earth’s Surface Processes: Sedimentary Rocks and Depositional Environments. |
j. Earth’s History Preserved in its Rocks: Stratigraphy and Geologic Time. |