Sensory Systems/Physiology of Pain
Introduction: What is Pain?
[edit | edit source]Pain is defined as "an unpleasant sensory and emotional experience associated with actual or potential tissue damage, or described in terms of such damage" by the International Association for the Study of Pain (IASP). Besides, pain is also a complex sensory pathway which is essential for survival. The mechanisms of the nervous system that detect stimuli having the potential to cause tissue damage are indispensable to trigger behavioural processes that protect the tissue against current or further damage. Amongst others, these stimuli include strong mechanical forces, temperature extremes and exposure to chemicals or oxygen deprivation.
Types of Pain
[edit | edit source]In general, pain can be divided in two categories: acute or chronic.
Typical characteristics of acute pain are a sudden onset and a duration of less than six months. Its underlying pathology can often be easily identified, since acute pain is well localized and defined through its association with tissue damage. Furthermore, acute pain normally subsides with tissue healing.
In contrast, chronic pain extends beyond normal recovery time and persists after the normal healing process occurred. It may or may not signal continued tissue damage, and is often associated with activation of dysfunctional neuro- logical or psychological response. Many patients suffering from chronic pain have a long history of treatment failures (i.e. medication was tried with no effects) and show common side effects such as depression, anger, frustration, decreased activity level or decreased sleep.
But pain can also be classified according to the sort of damage that causes it. The two main categories are then nociceptive pain caused by tissue injury and neuropathic pain, which arises due to nerve damage. Typical subcategories as well as characteristics and mechanisms are listed in Table 1. A widely discussed third category is psychogenic pain, which often has its origin either in tissue or nerve damage, but the pain caused by that damage is increased or prolonged by psychological factors such as fear, depression, stress, or anxiety. Cases where pain originates only from a psychological condition are rare.
Type of Pain | Characteristics | Mechanisms |
---|---|---|
Nociceptive
- Somatic (tissue injury) - Visceral - Inflammatory (musculoskeletal) |
Constant or cramping, poor localization Localized or diffuse pain, hyperalgesia, allodynia |
Visceral distension Associated with localized inflammation |
Neuropathic
- Causalgia (neuralgia, CNS lesions, ...) - Functional (FM, thalamic syndromes, ...) |
Diffuse deep pain, hyperalgesia, allodynia |
Dysregulation of exitatory or inhibitory mechanisms in CNS |
Nociceptive Signal Pathways
[edit | edit source]
(Based on ScoreUSMLE (2015)[2])
To understand the physiology of pain, the easiest way is to follow the nocicep- tive signal pathways from the sensory receptors to the brain. Special attention needs to be paid to the integration and modulation of the nociceptive signal at different steps in the Central Nervous System (CNS). While pain refers to the perception of a feeling, nociception (lat. nocere, "to hurt") refers to the sensory process that is triggered. Noxious stimuli such as strong mechanical forces or temperature extremes are detected by so-called nociceptors, which transduce these stimuli into electrical signals. The signals are then conducted through the primary afferent nerve fibres to the dorsal horn of the spinal cord. There, the primary neurons make a synaptic contact with the secondary neurons, which immediately cross in the spinal cord and then form the spinothalamic and spinoreticular tracts. Through these tracts the projections are sent to the thalamus, where the afferents subsequently make a second synapse. The tertiary neurons lead to the somatosensory cortices, which are involved in the sensory quality of pain including intensity, duration and location.
From the Periphery to the CNS
[edit | edit source]
(Based on Hasudungan (2013) [3])
A nociceptive stimulation initializes a cascade of events. First, a bunch of pro-nociceptive inflammatory substances including potassium, bradykinin, histamine and substance P will be released into the periphery near the injury (Table 2). These lead to hyperalgesia, i.e. an increased sensitivity to pain that can be measured as a lower pain threshold in and around the lesion.
Substance | Source |
---|---|
Potassium (K+) | Damaged cells |
Serotonin | Blood (platelets) |
Bradykinin | Blood (plasma) |
Histamine | Mast cells |
Prostaglandins | Damaged cells |
Leukotrienes | Damaged cells |
Substance P | Primary nerve afferents |
Next, free nerve endings called nociceptors will be activated by the noxious stimulus. They transduce the stimuli to electrical signals which will then be passed on through the afferent nerve fibers to the dorsal horn of the spinal cord.

(Based on Marchand (2008)[1])
The afferent fibers fall into three groups: Aβ-fibers, Aδ-fibers and C-fibers. Aβ-fibers usually conduct non-nociceptive signals, but are listed here since they do participate in pain modulation as described later. The characteristics of the different afferent fibers are listed in the table below:
Aβ-fibers | Aδ-fibers | C-fibers | |
---|---|---|---|
Location | Skin | Skin | Skin, muscle and visceral organs |
Diameter | 6 to 12 µm
myelinated |
1 to 5 µm
myelinated |
0.2 to 1.5 µm
unmyelinated |
Conduction | 35 to 75 m/s | 6 to 30 m/s | 0.5 to 3 m/s |
Role | Light touch, proprioception |
Temperature, Nociception |
Nociception (mechanical, thermal |
Increasing diameter and myelination boost the conduction velocity of the stimulus towards the spinal cord. The Aδ-fibers conduct the nociceptive signal relatively rapidly, which is why they are responsible for the nociceptive reflex (e.g. you pull away your hand very fast if you touch something hot) as well as the sharp localization of pain. In contrast, C-fibers have a slow conduction velocity (no myelination, small diameter) and therefore lead to a dull aching, non-localized and longer lasting pain.
Secondary Neurons in the Spinal Cord
[edit | edit source]
(Based on Hasudungan (2013)[3])
The afferent nerve fibers have a first synaptic contact with secondary neurons in the dorsal horn of the spinal cord. In addition, both non-nociceptive and nociceptive afferents have at this junction a synaptic interaction with an important network of exitatory interneurons and pain neurotransmitters including substance P or glutamate. These modulate the nociceptive signal before it is projected to superior centers of the CNS. The secondary neurons travel up the spinal cord along two main pathways: the spinothalamic and the spinoreticular tract. Both decussate to the contralateral side of the spinal cord within a few segments of the level of entry. While in the spinothalamic tract the secondary neurons ascend directly to the thalamus, the neurons in the spinoreticular tract synapse again in the reticular formation before leading to the thalamus. The reticular formation is located in the brainstem and is involved in a wide range of functions including attention and consciousness. Therefore it is believed to affect changes in the level of attention and the emotional reactions in response to pain.
From Nociception to Pain
[edit | edit source]Pain can only be experienced when nociceptive signals reach the cortex. Therefore, the neurons that come upwards along the spinal cord synapse a last time in the thalamus. From there, tertiary neurons guide the nociceptive information to different structures in the cortex: the somatosensory cortices (SI and SII) are mainly involved in the sensory discrimination of pain, while the anterior cingulate cortex (ACC) and the insular cortex (IC) are associated with the affective components of pain.
In the somatosensory cortices, both the nociceptive and the normal somatic
sensory information converge on the same cortical area. Therefore, information
on the location and the intensity of pain can be processed to become a
localized painful feeling. This cortical representation of the body is described
in Penfield’s homunculus.
Modulation of Pain Perception
[edit | edit source]Pain is a dynamic phenomenon perceived differently by every human being. The reason for this is, that the nociceptive signal is not only transmitted to the cortices but also modulated at multiple levels within the CNS. This modulation includes excitatory and inhibitory mechanisms from the brain stem, the autonomic nervous system, and the cortical structures responsible for the emotional (ACC and IC) as well as cognitive (SI and SII) aspect of pain.
Here, only inhibitory endogenous modulation mechanisms will be discussed. They are important to understand the possibilities to treat pain. To better understand their role, attention should be paid to three levels of modulation in the CNS (analogous to Marchand (2008)[1]):
- spinal mechanisms producing localized analgesia, i.e. insensibility to pain,
- descending inhibitory mechanisms from the brainstem producing diffuse inhibition of pain, and
- superior center effects that will either modulate descending mechanisms or change the perception of pain by reinterpreting the nociceptive signal.
Spinal Mechanisms: Gate Control Theory
[edit | edit source]
The gate control theory was developed by Melzack & Wall in 1965[5]. It suggests tha pain can be inhibited at the first synapse, i.e. between primary afferent fibers and secondary neurons at the spinal cord level. When C-fibers are firing, they do not only pass the noxious signal on to the secondary neuron, but also impede inhibitory interneurons. This is interpreted as the gate being closed and only noxious signals are forwarded. On the other hand, if Aβ-fibers are firing, they excite the inhibitory neurons. Therefore, the activation of the secondary neuron is not limited to nocious signals, which is interpreted as the gate being opened. Depending on the relative rates of firing between C-fibers and Aβ-fibers, the pain signal is blocked.
A well-known example of the gate control theory is, that pain sensation after
hitting your elbow decreases when you rub it. Its advantages are that it is not
noxious and no medication is needed, while it is leading to immediate pain
modulation. However, as soon as the high frequency stimulus that activates
the Aβ-fibers is gone (i.e. as soon as you stop rubbing you elbow), also the
pain level will not be diminished anymore.
Descending inhibitory Mechanisms: Endogenous Opioids
[edit | edit source]Already for a very long time it has been known that opium and its derivatives such as morphine and heroin are painkilling. In the 1960s and 1970s, their receptors were found, predominantely in the periaqueductal gray matter (PAG) and the rostroventral medulla (RVM) in the brainstem as well as in the spinal cord. This implied, that similar chemicals are produced by the nervous system to modulate pain endogenously. Serotonin and norepinephrine are the main transmitters of the descending inhibitory nerve system. These inhibitory pathways then recruit interneurons in the spinal cord to produce a pain-relieving response over the rest of the body, which is also known as counter-irritation. Examples for this kind of modulation are not only numerous painkillers, but also massage, acupressure, and acupuncture. In the latter two the endogenous system is triggered by additional pain, which is why they are usually only used after trying other options to modulate pain.
Superior Center Effects
[edit | edit source]
It has been shown, that cognitive manipulations such as distraction, hypnosis or expectation influence pain perception of individuals.[6]. Regarding distraction, a reduction in the activity of the cortical areas related to pain processing, such as the somatosensory cortex or the medial cingulate region of ACC, has been observed. This reduction would arise from the competition between attention to pain, which is a highly salient stimulus, and the conscious direction of attention towards another activity involving information processing, which constitutes distraction [7].
Furthermore, it has also been confirmed that suggestion of pain intensity can change the brain activity related to pain perception.[8] These results support the idea that placebo effect can adapt the performance of endogenous pain modulation. Later studies revealed an increase in the activity of the rostral anterior cingulate cortex (rACC) and its projections to the amygdala and the periaqueductal grey (PAG). This observation suggests that placebo effect originates at the rACC. Nevertheless, it would exert its influence over pain perception through the capability of PAG to modulate the activity of the noxious stimuli transmitting neurons. The influence of the amygdala on this circuit would derive from its role in the emotional interpretation of stimuli. It would allow for the modulation of pain through the unconscious process of conditioning, and not only via the conscious expectation originated at rACC [9].
Phantom Limb Pain
[edit | edit source]History
[edit | edit source]The phenomenons known as phantom limb pain and phantom limb sensation have been known since the XVI century. A French military surgeon, Ambroise Paré, was the first person to medically document phantom limb pain and phantom limb sensation in 1551:

“For the patients, long after the amputation is made, say they still feel pain in the amputated part. Of this they complain strongly, a thing worthy and almost incredible to people who have not experienced this”. [10]
A Scottish neurologist, Charles Bell, published in 1830 a description of the phenomenon in the Nervous System of the Human Body. However, It was not until 1871 that the phrase “phantom limb pain” was introduced by Silas Weir Mitchell, an American military surgeon. He gave the first modern description of the post surgical “ghost” feeling that many amputees experience:

“There is something almost tragical, something ghastly, in the notion of these thousands of spirit limbs haunting as many good soldiers, and every now and then tormenting them... when... the keen sense of the limb’s presence betrays the man into some effort, the failure of which of a sudden reminds him of his loss”. [10]
Differences between phantom limb sensation and phantom limb pain
[edit | edit source]Phantom limb pain and sensation are inextricably linked. However, they are not the same and it is therefore important to distinguish them from one another.
Phantom limb sensations include kinesthetic sensations such as feelings of length, girth or posture of the limb, kinetic sensations such as spontaneous or intentional movements, and exteroceptive sensations such as tingling, itching or pressure [11]. These sensations can appear either after amputation, congenital limb deficiency, or when the sensation in the limb is gone, for instance due to spinal cord injury [10] . Studies have shown that phantom limb sensations are experienced by 90-98% of all patients. [12] [13] [14] A special phenomenon that is experienced by approximately 25-40% of the patients with phantom sensations is telescoping, the feeling that the phantom limb is retracting over time towards the stump, until it is attached to it or inside it. [14] [15]
Phantom limb pain is variable and can be described as burning, stabbing, or throbbing sensations, among others. [16] Moreover, very complex pain sensations can occur, for example the feeling of twisting in the phantom limb, or of fingernails digging painfully into the palm of the phantom hand. [11] The majority of individuals experience phantom limb pain after amputation, with a prevalence ranging from 55 to 85%. [17][10] In many cases there is pain at the stump as well as in the phantom limb, making it difficult to distinguish one from the other.
Studies have also shown that almost all amputees who experience phantom limb sensation also suffer from phantom limb pain; the opposite is rarely the case. [10]
Phantom Limb Sensation | Phantom Limb Pain | |
---|---|---|
Symptomatology | Kinestetics and kinetic sensations. | Burning, stabbing, or throbbing sensations, among others |
Origin | Amputation, congenital limb deficiency or limb sensitivity disappearance. | Amputation |
Co-manifestation | Mostly manifested along with phantom limb pain. | Rarely manifested along with phantom limb sensation. |
Epidemiology
[edit | edit source]Over the last decades many clinical studies on phantom limb pain and sensation have been carried out, outlining some common tendencies among patients suffering from the syndrome.
The prevalence of phantom limb pain has been shown to vary with the level of the amputation: the higher the level of the amputation, the greater the incidence of moderate to severe phantom limb pain. [10] There are also hints that the incidence of pain may be lower in the case of a planned surgical amputation of a non-painful limb, than in traumatic loss of a phantom limb or one that had pre-existing pain. [12] Furthermore, the pain is usually felt in the most distal part of the phantom limb, such as the fingers in the hands or toes in legs. It has been speculated that this is due to the fact that there is a greater representation of limb endings in the somatosensory cortex. [13] [14]Interestingly, the prevalence of phantom pain in lower limb amputees is much higher that in upper limb amputees. [10]
Regarding frequency, phantom pain is continuously present in a subset of patients, while in others it tends to occur episodically in the form of pain attacks. [18] However, even in these cases, a continuous pain can also exist in between. The pain attacks can last from a few seconds to up to several hours. In rare cases, patients can experience the episodes over several days.[17]

Phantom pain usually starts as early as the first few days to weeks after the amputation but it can also start even years after, triggered by operations in the stump. [17] The pain can be persistent many years after the amputation. However, different clinical studies disagree on how long the pain persists in a phantom limb. For example, studies on elderly patients who lost their limbs as a result of vascular insufficiency have yielded different results than studies on younger amputees with traumatic amputation following injury. [10] Nevertheless, several studies reported common tendencies: only 16% of people that had phantom pain no longer suffer from it, while in another 37% there had been a significant reduction, 44% suffered from the same pain, and in 3% of the cases the pain had become stronger over time. [19] [17] Another common benchmark is that if the pain is still persistent six months after the amputation it becomes very difficult to treat. [20]
Interestingly, many patients often complain that the pain that had existed in their limbs prior to amputation persists in the phantom limb. This persistence of pain could be a form of sensory memory. [21]Related to these findings, it has also been reported that phantom limb sensations are less common in young children than in adults. A possible explanation is that in young children there has not been enough time for the body image to consolidate. In fact, according to some studies, the incidence of phantom sensations increases drastically with the age of the children. [12]
According to a study performed on 139 patients [18], phantom pain can be triggered by [17] :
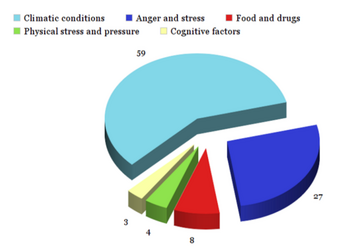
- Climatic conditions: 59%. This includes a 55% due to weather, 1% due to the season and 3% due to the ambient temperature.
- Anger and stress: 27%
- Food / drugs: 8%
- Physical stress and pressure: 4%
- Cognitive Factors: 3%. Examples of cognitive factors are fixing attention on the phantom limb and observing the injury.
The reason why these factors trigger phantom pain will become clear in the next section.
Origin of phantom pain
[edit | edit source]In accordance with the fact that phantom pain is considered a multifactorial pain syndrome, and current theories on the pathophysiology of phantom limb pain are usually separated into peripheral, central and psychological components. These three areas are strongly interconnected.
Peripheral mechanisms
[edit | edit source]After amputation cellular differentiation takes place, leading to the degeneration of the distal peripheral nerves as well as the development of neuromas - which are growths or tumors in nerve tissues. [22]

Neuromas are formed from the regeneration of the surviving proximal nerve section and can generate abnormal discharges that cause the persistence of the pain. In fact, in several studies, evidence of hyperactivity in the stump muscles was found when compared to the contralateral limb. [23] [13] This hyperactivity was present in the form of slight twitches in small fiber bundles or even spasmodic contractions of the entire stump.
The fact that pain is not eliminated by conduction blockage of peripheral nerves, as well as the fact that pain can still persist even after ectopic discharges in the peripheral nerves are eliminated through pharmacotherapy or surgery, proves that phantom pain is a complex mechanism not solely due to the development of neuromas. [21] In fact, there are some additional peripheral mechanisms involved in phantom pain: blood circulation effects and sympathetic activation.
a) Blood circulation effects
[edit | edit source]Under low temperatures our sympathetic nervous system is stimulated, causing vasoconstriction in skin, arms and legs. [24] This in consequence leads to hypoperfusion in the stump, which can increase the activity of nociceptive nerve fibers and thus reinforce the sensation of pain. This is due to both ischemia and the cooling of neurons. [18]These vascular mechanisms are the reason why weather is one of the main triggers of phantom pain.
Furthermore, a connection between brief, strong increases in muscle tension in the stump and subsequent phantom pain episodes has been established in several studies. [24] Mechanical pressure and strong muscle contraction may also have perfusion effects, which in turn could aggravate the pain. Moreover, perfusion effects may also appear as a consequence of some foods and drugs like coffee, explaining their pain triggering nature.
b) Sympathetic activation
[edit | edit source]Within neuromas sympathetic efferent nerve endings can become entangled with nociceptive afferent nerves, directly interacting and resulting in an increased sympathetic activity and worsening of the phantom pain. [25]
Central nervous factors
[edit | edit source]As explained before, phantom pain is not caused solely by peripheral mechanisms. The complexity of the sensations in the phantom limb also suggests that these perceptions involve higher nervous structures. [11]
a) The neuromatrix theory
[edit | edit source]The main theory to explain phantom pain in the context of the central nervous system is the neuromatrix theory. The neuromatrix includes the cortex, especially the primary somatosensory cortex and the posterior parietal lobe, the thalamus, and the limbic system.

This theory postulates that the representation of the body in the nervous system is being constantly modified by sensory inputs, creating a neurosignature. After the removal of a body part, the persistence of the neuromatrix creates painless sensations on the phantom limb, while pain comes from an abnormal reorganisation within the neuromatrix. More specifically, the neuromatrix continues to send an outgoing signal after the amputation, and the alteration of these signals may be responsible for pain. In theory, it could be possible to decrease the pain by eliminating the outgoing signal from the neuromatrix, or by creating a normal return signal via electrical stimulation. In fact, some studies indicate that phantom pain can be linked to neural activity and function of the thalamic representation of the amputated limb. This can be shown by the fact that microstimulation in the ventrocaudal thalamus produces a painful sensation in the phantom limb. [21]
While the neuromatrix hypothesis can be useful for describing the origin of pain, it does not explain why relief of phantom sensation rarely alleviates phantom pain, why the sensation can suddenly go away, or why not all amputees experience phantom limb pain. [21] Therefore, other possible explanations must be taken into consideration.
b) Central hyper-responsiveness
[edit | edit source]Several studies have demonstrated that after amputations and other types of nerve damage, a state of hyperexcitability in the central nervous system may occur. [23] [13]
Cortical Reorganization
[edit | edit source]In addition to the hyper-responsiveness, and possibly as a consequence, after an amputation changes in the cortical representation of the body, take place. These changes are generally observed after deafferentation, and are particularly pronounced in the primary somatosensory cortex.

Several studies in both primates and humans have found that months after an amputation the representation of the body-parts adjacent to it in the primary somatosensory cortex had expanded a few millimeters, and almost completely occupied the former territory of the amputated part. [26] [27] [28] [29] Interestingly, the area over which this reorganization takes place is more variable in lower limb amputees. This cortical reorganization can even be observed as temporary shifts during local anesthesia. [30] [31] and also in the opposite way as a consequence of intensive training of a body part. [32] It also happens in the motor cortex, probably as a result of the strong connections between the somatosensory and motor cortex. [33] [34] Intuitively, it could be thought that this rearrangement would be beneficial, since an otherwise unused area of the cortex can thus be dedicated to an actual part of the body, eliminating the lost limb from the body scheme and thereby reducing phantom pain. But this reorganization comes at a cost, and, the existence of a positive relationship between the extent of reorganization and intensity of the phantom pain has been observed. [29] [35]These results have led to the belief that cortical reorganization is in fact maladaptive plasticity and one of the causes of phantom pain. [36]
Cortical reorganization is mediated through three fundamental mechanisms:
a) Cortical processes
[edit | edit source]For short-term reorganization, the main mechanism is the unmasking of existing latent connections between adjacent areas. [31] It is assumed that this mechanism is triggered by a change in the equilibrium between excitatory and inhibitory influx. [37] [30] However, for long-term reorganization processes, new neural connections form. Hence, in this case structural changes take place in addition to the functional ones. [18]
b) The spinal processes
[edit | edit source]In case of heavy or prolonged nociceptive stimulation the previously mentioned central sensitization may occur also in the dorsal horn neurons of the spinal cord. This can lead to an increased spontaneous activity and increased responsiveness to non-noxious stimuli [38] [39], thus prolonging the pain long after the noxious stimuli are present.
So far all findings on amputation-induced changes in the spinal cord all come from animal experiments. They indicate that afferent fibers coming from the stump extend into the deafferentiated area in the spinal cord and brainstem. [40] [10]
Insight into the role of spinal neurons in the generation of pain has also been obtained from the study of burn victims. These studies indicate that after a burn injury, spinal cord neurons increase their discharge rates , resulting in the hyperexcitability of the neuron. This hyperexcitability was not affected by local anaesthetic blocks on the injured site. This indicates that a sensitization or ‘pain memory’ had already been created. Applied to patients with amputations, this suggest that blocking the spinal cord before and during surgical amputation may help reduce or prevent this sensitization process in the spinal cord. [21]
Phantom limb pain can also be experienced by people with complete spinal cord injury, indicating that in addition to the peripheral and spinal processes, also changes in the central nervous system can be involved in the generation of pain. [10]
c) Thalamic processes
[edit | edit source]Apart from the deep-brain stimulations, which have revealed thalamic contributions to phantom pain, not very much is know about the role of the thalamus in this context. Animal experiments with local anesthesia have reported short-term and reversible reorganization of the representation of some body parts in the thalamus. [41]
Psychological factors
[edit | edit source]Both stress and anger are also considered triggering factors for phantom pain, as discussed in the epidemiology section. In fact, a consistent and overall tight positive correlation between stress and phantom pain was found in 74% of subjects of a study. [18] This is probably due to the fact that both psychological and physical stress have a nociceptive effect that include physiological mechanisms such as sympathetic activation, vasoconstriction and muscle tension, which are all triggering mechanisms. Many patients have also reported that phantom pain can be enhanced or even triggered consciously, and reversely, that it diminishes by distraction. [11] [12]
Treatment of phantom pain
[edit | edit source]Phantom limb pain still lacks effective treatment. Some of the approaches used are: analgesics -with limited effect-, neuroleptics, antidepressants, nerve blocks, stimulation of the nervous system -specially the spinal cord and the nerves in the stump but also deep brain stimulation-, vibration therapy and massages on the stump, heat application, biofeedback and acupuncture. In some cases scar tissue entangling a nerve is removed surgically. [42] [43] Another popular therapy is the use of a mirror to allow the patient to “see” the phantom limb, by observing the reflection of the remaining limb. As in the case of the other therapies, it is not totally effective and is unsuccessful for many patients. In fact, about 40% of people do not benefit from mirror therapy. [44]
Pain Treatment
[edit | edit source]Nonsteroidal anti-inflammatory drugs (NSAID)
[edit | edit source]NSAIDs are used to treat mild to moderate pain, such as headache, sprains or toothache. NSAIDs are anti-inflammatory, which makes them also effective for treating injuries or conditions such as arthritis and postoperative pain. NSAIDs work by the function of COX-2 inhibitors. They directly target cyclo-oxygenase-2 (COX-2), an enzyme that produces prostaglandin which is responsible for inflammation and pain. They have also been shown to have positive effects in reducing the occurrence of cancers and pre-cancerous growths as well as in suppressing inflammatory neurodegenerative pathways in mental illnesses as in depression and schizophrenia. [45] [46] However, they have severe side effects. NSAIDs significantly increase heart attacks and strokes.
To the class of NSAIDs, inhibiting COX-2 and thus the production of prostaglandin, belong a range of medications:
Ibuprofen
[edit | edit source]
Ibuprofen belongs to the group of the NSAIDs. It stops the production of prostaglandins by inhibiting Cyclo-oxygenase COX-1 and COX-2. It is used to treat pain, fever and inflammations. Its half-life is between 1 and 3 hours.[47]
Naproxen
[edit | edit source]
Naproxen is a NSAID that is used to treat pain, fever and inflammations and is with 8-12 hours relatively long active.[48]
Aspirin®
[edit | edit source]
Acetylsalicylic acid is a NSAID that belongs to the group of the salicylates. It treats pain, fever and inflammations and inhibits the aggregation of thrombocytes. It's mechanism is according to the one of the NSAID’s. Acetylsalicylic acid is used to treat fever and pain of different causes. Usually, it is used to treat acute pains like headache, toothache, pain coming from joints, tendons or the back, but also fever and pain from a cold. It can also be used to prevent the formation of thromboses when used daily in low doses.[49]
Paracetamol
[edit | edit source]
Paracetamol is also known as Dafalgan ® or Panadol ®. It is derived from Acetanilid, which was in 1880 one of the first medications against fever that was launched. Paracetamol is used to alleviate pain and reduce fever. In contrast to the NSARs, it doesn't combat inflammations and does not inhibit the aggregation of thrombocytes. However, it is also involved in the blocking of the prostaglandin synthesis. Its half-life period is about 2 to 3 hours, which is rather short. Its effect lasts around 4 to 6 hours. Possible application areas are: headache, toothache, muscular and joint pain, pain after surgery or in context with fever and flue.[50]
Metamizole
[edit | edit source]
Metamizole is a (not acidic) analgesic and belongs to the group of the pyrazolones. It is a prodrug which in the body becomes biotransformed into different active metabolites. Metamizole can be used to treat pain, fever and spasms. Because of its spasmolytic properties, it is often used to treat colics. Its mechanism is not yet fully understood. Possible drug targets are cyclo-oxygenases and cannabinoid receptors.[51]
Opioids
[edit | edit source]Opioids play an essential role in pain management especially for moderate to severe pain. They are used for acute pain, in palliative care and pain treatment of degenerative conditions. Morphine is the prototypical opioid. Opioids interact with opioid receptors which are G-protein coupled receptors that are mostly found in the brain and spinal cord but also the gastrointestinal system and in other tissue. By binding of the opioid to the opioid receptor the Gprotein is activated which leads to inhibitory effects on cAMP production, Ca2+ influx and K+ efflux that result in hyperpolarization of the cell and reduction of neuronal excitability. In the spinal cord opioids inhibit the release of substance P from primary sensory neurons reducing the transfer of painful sensations to the brain. In the brainstem nociceptive transmission is modulated by opioids.[52]
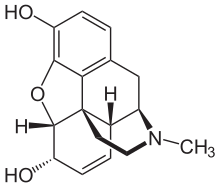
Antidepressants
[edit | edit source]Antidepressants are used in the treatment of neuropathic pain and fibromyalgia. The analgesic effect does also show in non-depressed patients, so they do not only work by improving a depressive state that can accompany chronic pain. Examples for antidepressants with analgesic effects are tricyclic antidepressants (TCA) and serotonin noradrenaline reuptake inhibitors (SNRI). Antidepressants have no nociceptive effect but act on the locus coeruleus and bind to noradrenaline, which is involved in intrinsic control of pain. The exact mechanism, however, is still unclear.[53]
Antiepileptics
[edit | edit source]Antiepileptic drugs are used for treatment of neuropathic pain. There are several different ways antiepileptics relieve neuropathic pain. For example, older antiepileptic drugs such as phenytoin and carbamazepine which is mainly used for trigeminal neuralgia, work by reducing neuronal excitability by means of frequency-dependent blockade of sodium channels.[54]
Alternative Treatments
[edit | edit source]Deep Brain Stimulation
[edit | edit source]

Deep brain stimulation (DBS) has been used for the treatment of chronic pain since the early 1970s. DBS shows favorable results for the treatment of chronic pain, including failed back surgery syndrome (FBSS), phantom limb pain, and peripheral neuropathic pain with a higher response rate for those with nociceptive pain compared to neuropathic pain. It also shows promising results in cephalgia, with cluster headaches carrying the best success rates.[55] Nevertheless, the capability of DBS to relieve pain in a very long-term is reduced by the increasing tolerance to its application over time. This can be specially critical for the treatment of chronic pain. Possible solutions are the periodical deactivation of the DBS to reduce tolerance or the usage of adaptive DBS systems in closed-loop.
A crucial point for the development of the second possibility is the identification of useful biomarkers to regulate stimulation. Three main strategies are followed to meet this goal. First, monitoring neural activity in different brain areas during or after the delivery of a pain-relieving stimulation can help identify which of them are more or less active during an efficient stimulation. Then, the DBS system can try to duplicate that same activity to induce pain relief. Second, spectral analysis of neuronal activity in the application area could help predict the effectivity of the signal. Third, the simultaneous recording of neural activity in different brain areas allows for the conditioning of the stimulus on the functional brain connectivity at that moment. [56].
The pathways by which DBS has an effect on pain are still not completely understood. Nevertheless, several trials help formulate different hypotheses. When DBS is applied on the ventral posterior lateral (VPL), ventral posterior medial thalamus (VPM), periventricular grey (PVG) or periaqueductal grey (PGA), low-frequency signals (≤50 Hz) lead to analgesic effects, while high-frequency ones (>70 Hz) provoked hyperalgesia. Thus, it is hypothesised that the analgesic effect of DBS could be derived from the potentiation of joint low-frequency neural activity or the disruption of high-frequency one. [57].
Cannabinoids
[edit | edit source]In some states, marijuana can be medically prescribed. It often comes to use as a treatment for chronic pain when no other drug is available. The effect of marijuana is mediated through the cannabinoid receptors CB1 and CB2. Still, scientific evidence is missing as the regulations for testing marijuana in people are very strict. Getting the clearance to work with THC is almost impossible. Allowing research on marijuana would help to identify the compound or combination of compounds that cause the adverse effects and develop a potent, effective and safe new drug.[58]
To get around the restrictions, some scientists are investigating an alternative: the marijuana-like compounds produced naturally in the brain called endocannabinoids. They act on the neurons in a similar way as the cannabinoids and help regulate pain, appetite and anxiety. When molecules bind to the CB1 receptor, it leads to pain relief. Research is also done in the usage of positive allosteric modulators, PAMs, that increase the odds a signaling molecule, in this case endocannabinoid, will find its receptor, which is here the CB1 receptor. That way, PAM would only be boosting endocannabinoid signaling under conditions in which it's already being released.[59]
References
[edit | edit source]- ↑ a b c d Marchand, S. (2008), ‘The physiology of pain mechanisms: From the peripheryto the brain’, Rheumatic Disease Clinics of North America 34(2), 285-309.
- ↑ ScoreUSMLE (2015), ‘Spinal pathways made super easy (part 1-2)’, Youtube Video, (accessed 21.7.2015).
- ↑ a b Hasudungan, A. (2013), ‘Nociceptors - an introduction to pain’ (Explains the ascending pathway, very easy to understand), Youtube Video, (accessed 21.7.2015).
- ↑ Patel, N. B. (2010), ‘Physiology of pain’, Extracted from Kopf and Patel (Ed) „Guide to Pain Management in Low-Resource Settings“, 2010, International Association for the Study of Pain.
- ↑ Melzack, R. & Wall, P. D. (1965), ‘Pain mechanisms: a new theory’, Science 150, 971--979.
- ↑ Apkarian, A. V., Bushnell, M. C., Treede, R. D. & Zubieta, J. K. (2005), ‘Human brain mechanisms for pain perception and regulation in health and disease’, European Journal of Pain 9(4), 463-484.
- ↑ Malcom H. and Johnson MA (2005). "How Does Distraction Work in the Management of Pain". Current Pain and Headache Reports. 9: 90–95.
- ↑ Goffaux, P., Redmond, W. J., Rainville, P. & Marchand, S. (2007), ‘Descending analgesia – when the spine echoes what the brain expects’, Pain 130(1-2), 137-143.
- ↑ U. Bingel, J. Lorenz, E. Schoell, C. Weiller, C. Büchel (2006). "Mechanisms of placebo analgesia: rACC recruitment of a subcortical antinociceptive network". Pain. 120: 8–15.
{{cite journal}}
: CS1 maint: multiple names: authors list (link) - ↑ a b c d e f g h i j C. M. Kooijman, P. U. Dijkstra, J. H. B. Geertzen, A. Elzinga, and C. P. Van Der Schans, “Phantom pain and phantom sensations in upper limb amputees: An epidemiological study,” Pain, vol. 87, no. 1, pp. 33–41, 2000.
- ↑ a b c d P. Jensen, T.S. & Rasmussen, Phantom pain and other phenomena, Textbook o. Edinburgh: Churchill Livingstone, 1994.
- ↑ a b c d e V. S. Ramachandran and W. Hirstein, “The perception of phantom limbs. The D. O. Hebb lecture,” Brain, vol. 121, no. 9, pp. 1603–1630, 1998.
- ↑ a b c d B. Cronholm, Phantom limbs in amputees: a study of changes in the integration of centripetal impulses with special reference to referred sensations. Stockholm, 1951.
- ↑ a b c E. Frank, B. & Lorenzoni, “Phantomerleben und Phantomschmerz. Fortschritte der Neurologie und Psychiatrie,” vol. 60, pp. 74–85, 1992.
- ↑ L. Schmalzl, “‘Pulling telescoped phantoms out of the stump’: Manipulating the perceived position of phantom limbs using a full-body illusion,” Front. Hum. Neurosci., vol. 5, no. November, pp. 1–12, 2011
- ↑ K. Sherman, R.A., Katz, J., Marbach, J.J. & Heermann-Do, Locations, characteristics, and descriptions. New York: Plenum Press, 1997.
- ↑ a b c d e M. Döbler, K. & Zenz, “Stumpf- und Phantomschmerz,” in Lehrbuch der Schmerztherapie, Stuttgart: Wissenschaftliche Verlagsgesellschaft, 1993, pp. 377–384.
- ↑ a b c d e C. Winter-Barnstedt, “Phantomschmerz nach Extremitätenamputation : Diagnostik und Biofeedback-Behandlung,” Ruprecht-Karls-Universität Heidelberg, 2001.
- ↑ S. W. Wartan, W. Hamann, J. R. Wedley, and I. McColl, “Phantom pain and sensation among British veteran amputees.,” Br. J. Anaesth., vol. 78, pp. 652–659, 1997.
- ↑ C. Sherman, RA, Sherman, “Prevalence and characteristics of chronic phantom limb pain among American veterans: results of a trial survey,” Am. J. Phys. Med., vol. 62, no. 5, pp. 227–238, 1983.
- ↑ a b c d e R. G. Bittar, S. Otero, H. Carter, and T. Z. Aziz, “Deep brain stimulation for phantom limb pain.,” J. Clin. Neurosci., vol. 12, no. 4, pp. 399–404, 2005.
- ↑ “Neuroma,” Wikipedia, 2016. [Online]. Available: Neuroma [Accessed: 05-Jun-2016].
- ↑ a b W. Larbig, P. Montoya, H. Flor, H. Bilow, S. Weller, and N. Birbaumer, “Evidence for a change in neural processing in phantom limb pain patients.,” Pain, vol. 67, no. 2–3, pp. 275–283, 1996.
- ↑ a b R. A. Sherman, V. D. Griffin, C. B. Evans, and A. S. Grana, “Temporal relationships between changes in phantom limb pain intensity and changes in surface electromyogram of the residual limb,” Int. J. Psychophysiol., vol. 13, no. 1, pp. 71–77, 1992.
- ↑ R. A. Sherman, J. G. Arena, C. J. Sherman, and J. L. Ernst, “The mystery of phantom pain: Growing evidence for psychophysiological mechanisms,” Biofeedback Self. Regul., vol. 14, no. 4, pp. 267–280, 1989
- ↑ M. M. Merzenich, R. J. Nelson, M. P. Stryker, M. S. Cynader, A. Schoppmann, and J. M. Zook, “Somatosensory cortical map changes following digit amputation in adult monkeys.,” J. Comp. Neurol., vol. 224, no. 4, pp. 591–605, 1984.
- ↑ T. P. Pons, E. G. Preston, A. K. Ommaya, H. K. Jon, E. Taub, and M. Mishkin, “Massive Cortical Reorganization After Sensory Deafferentation in Adult Macaques,” Science (80-. )., vol. 252, no. 5014, pp. 1857–1860, 1991.
- ↑ T. Elbert, H. Flor, N. Birbaumer, S. Knecht, S. Hampson, W. Larbig, and E. Taub, “Extensive reorganization of the somatosensory cortex in adult humans after nervous system injury.,” Neuroreport, vol. 5, no. 18, pp. 2593–2597, 1994.
- ↑ a b H. Flor, T. Elbert, S. Knecht, C. Wienbruch, C. Pantev, N. Birbaumer, W. Larbig, and E. Taub, “Phantom-limb pain as a perceptual correlate of cortical reorganization following arm amputation.,” Nature, vol. 375, no. 6531, pp. 482–484, 1995.
- ↑ a b H. Buchner, C. Kauert, and I. Radermacher, “Short-term changes of finger representation at the somatosensory cortex in humans,” Neurosci. Lett., vol. 198, no. 1, pp. 57–59, 1995.
- ↑ a b P. M. Rossini, G. Martino, L. Narici, A. Pasquarelli, M. Peresson, V. Pizzella, F. Tecchio, G. Torrioli, and G. L. Romani, “Short-term brain ‘plasticity’ in humans: transient finger representation changes in sensory cortex somatotopy following ischemic anesthesia,” Brain Res., vol. 642, no. 1–2, pp. 169–177, 1994.
- ↑ W. M. Jenkins, M. M. Merzenich, M. T. Ochs, T. Allard, and E. Guic-Robles, “Functional reorganization of primary somatosensory cortex in adult owl monkeys after behaviorally controlled tactile stimulation,” J. Neurophysiol., vol. 63, no. 1, pp. 82–104, 1990.
- ↑ A. Karl, N. Birbaumer, W. Lutzenberger, L. G. Cohen, and H. Flor, “Reorganization of motor and somatosensory cortex in upper extremity amputees with phantom limb pain.,” J. Neurosci., vol. 21, no. 10, pp. 3609–18, 2001.
- ↑ R. Chen, B. Corwell, Z. Yaseen, M. Hallett, and L. G. Cohen, “Mechanisms of cortical reorganization in lower-limb amputees.,” J. Neurosci., vol. 18, no. 9, pp. 3443–50, 1998.
- ↑ H. Flor, T. Elbert, W. Mühlnickel, C. Pantev, C. Wienbruch, and E. Taub, “Cortical reorganization and phantom phenomena in congenital and traumatic upper-extremity amputees,” Exp. Brain Res., vol. 119, no. 2, pp. 205–212, 1998.
- ↑ E. a Franz and V. S. Ramachandran, “Bimanual coupling in amputees with phantom limbs.,” Nat. Neurosci., vol. 1, no. 6, pp. 443–444, 1998.
- ↑ M. Calford and R. Tweedale, “Immediate and chronic changes in responses of somatosensory cortex in adult flying-fox after digit amputation.,” Nature, vol. 332, no. 6163. pp. 446–448, 1988.
- ↑ L. Arendt-Nielsen and S. Petersen-Felix, “Wind-up and neuroplasticity: is there a correlation to clinical pain?,” Eur. J. Anaesthesiol. Suppl., vol. 10, pp. 1–7, 1995.
- ↑ J. Li, D. A. Simone, and A. A. Larson, “Windup leads to characteristics of central sensitization,” Pain, vol. 79, no. 1, pp. 75–82, 1999.
- ↑ S. L. Florence and J. H. Kaas, “Large-scale reorganization at multiple levels of the somatosensory pathway follows therapeutic amputation of the hand in monkeys.,” J. Neurosci., vol. 15, no. 12, pp. 8083–8095, 1995.
- ↑ M. A. Nicolelis, R. C. Lin, D. J. Woodward, and J. K. Chapin, “Induction of immediate spatiotemporal changes in thalamic networks by peripheral block of ascending cutaneous information.,” Nature, vol. 361, no. 6412, pp. 533–6, 1993.
- ↑ “Phantom limb,” Wikipedia, 2016. [Online]. Available: w: Phantom_limb Phantom_limb [Accessed: 05-Jun-2016].
- ↑ “Pain Management Health Center,” WebMD, 2015. [Online]. Available: phantom-limb-pain| [Accessed: 05-May-2016].
- ↑ J. Foell, R. Bekrater-Bodmann, M. Diers, and H. Flor, “Mirror therapy for phantom limb pain: Brain changes and the role of body representation.,” Eur. J. Pain, pp. 1–11, 2013.
- ↑ COX-2 Inhibitors and Cancer: Questions and Answers.” 2004. National Cancer Institut. 2004. http://www.cancer.gov/cancertopics/factsheet/APCtrialCOX2QandA.
- ↑ Muller, Norbert. 2010. “COX-2 Inhibitors as Antidepressants and Antipsychotics: Clinical Evidence.” Current Opinion in Investigational Drugs (London, England : 2000) 11 (1). England:31–42.
- ↑ “Ibuprofen.” 2017. PharmaWiki. https://www.pharmawiki.ch/wiki/index.php?wiki=ibuprofen.
- ↑ "Naproxen.” 2017. PharmaWiki. https://www.pharmawiki.ch/wiki/index.php?wiki=metamizol.
- ↑ “Acetylsalicylsäure.” 2017. PharmaWiki. https://www.pharmawiki.ch/wiki/index.php?wiki=Acetylsalicylsäure.
- ↑ “Paracetamol.” 2018. PharmaWiki. https://www.pharmawiki.ch/wiki/index.php?wiki=paracetamol.
- ↑ “Metamizol.” 2017. PharmaWiki. https://www.pharmawiki.ch/wiki/index.php?wiki=metamizol.
- ↑ Hemmings, H. C., & Egan, T. D. (2013). Opioid agonists and antagonists. In Pharmacology and physiology for anesthesia: Foundations and clinical application. Philadelphia, PA: Elsevier/Saunders.
- ↑ Obata, H. (2017). Analgesic Mechanisms of Antidepressants for Neuropathic Pain. International Journal of Molecular Sciences, 18(11), 2483. doi:10.3390/ijms18112483.
- ↑ Ryder, S., & Stannard, C. F. (2005). Treatment of chronic pain: Antidepressant, antiepileptic and antiarrhythmic drugs. Continuing Education in Anaesthesia Critical Care & Pain,5(1), 18-21. doi:10.1093/bjaceaccp/mki003.
- ↑ Falowski, Steven M. 2015. “Deep Brain Stimulation for Chronic Pain.” Current Pain and Headache Reports 19 (7). United States:27. doi:10.1007/s11916-015-0504-1.
- ↑ Shirvalkar, P., Sellers, K. K., Schmitgen, A., Prosky, J., Joseph, I., Starr, P. A., & Chang, E. F. (2020). "A Deep Brain Stimulation Trial Period for Treating Chronic Pain". Journal of Clinical Medicine. 9 (10): 3155.
{{cite journal}}
: CS1 maint: multiple names: authors list (link) - ↑ Alamri, A. and A.C. Pereira, E. (2022). "Deep Brain Stimulation for Chronic Pain". Neurosurgery Clinics of North America. 33 (3): 311–321.
{{cite journal}}
: CS1 maint: multiple names: authors list (link) - ↑ Fields, Douglas. 2014. “The Absurdity of ‘ Medical Marijuana .’” BrainFacts.org. 2014. http://www.brainfacts.org/thinking-sensing-and-behaving/diet-and-lifestyle/2014/the-absurdity-ofmedical-marijuana.
- ↑ Burrell, Teal. 2017. “Smoking Out the Therapeutic Possibilities of Cannabinoids.” BrainFacts.org. 2017. http://www.brainfacts.org/thinking-sensing-and-behaving/diet-and-lifestyle/2017/smoking-out-thetherapeutic-possibilities-of-cannabinoids-42017.