Sensory Systems/Spiders
Spider´s Visual System
[edit | edit source]Introduction
[edit | edit source]While the highly developed visual systems of some spider species have been subject to extensive studies for many decades, terms like animal intelligence or cognition were not usually used in the context of spider studies. Instead, spiders were traditionally portrayed as rather simple, instinct driven animals (Bristowe 1958, Savory 1928), processing visual input in pre-programmed patterns rather than actively interpreting the information received from their visual apparatus towards appropriate reactions. While Although this still seems to be the case in a majority of spiders, which primarily interact with the world through tactile sensation rather than by visual cues, some spider species have shown surprisingly intelligent use of their eyes. Considering its limited dimensions within the body, a spider´s optical apparatus and visual processing perform extremely well.[1] Recent research points towards a very sophisticated use of visual cues in a spider´s world when investigating topics such as the complex hunting schemes of the vision-guided jumping spiders (Salticidae) taking huge leaps of up to 30 times their own body length onto prey or a wolf spider´s (Lycosidae) ability to visually recognize asymmetries in potential mates. Even in the case of the night-active Cupiennius salei (Ctenidae), relying primarily on other sensory organs, or the ogre-faced Dinopis hunting at night by spinning small webs and throwing them at approaching prey, the visual system is still highly developed. Findings like these are not only fascinating but are also inspiring other scientific and engineering fields such as robotics and computer-guided image analysis.
General structure of a spider´s anatomy
[edit | edit source]
A spider´s anatomy primarily consists of two major body segments, the prosoma and the opisthosoma, which are also known as the cephalothorax and abdomen, respectively. All extremities as well as the sensory organs including the eyes are located in the prosoma. Other than the visual system of arthropods featuring compound eyes, modern arachnid eyes are ocelli (simple eyes consisting of a lens covering a vitreous fluid-filled pit with a retina at the bottom), of which spiders have six or eight, characteristically arranged in three or four rows across the prosoma´s carapace. Overall, 99% of all spiders have eight eyes and of the remaining 1% almost all have six. Spiders with only six eyes lack the “principal eyes”, which are described in detail below.
The pairs of eyes are called anterior median eyes (AME), anterior lateral eyes (ALE), posterior median eyes (PME), and posterior lateral eyes (PLE). The large principal eyes facing forward are the anterior median eyes, which provide the highest spatial resolution to a spider, at the cost of a very narrow field of view. The smaller forward-facing eyes are the anterior lateral eyes with a moderate field of view and medium spatial resolution. The two posterior eye pairs are rather peripheral, secondary eyes with wide field of view. They are extremely sensitive and suitable for low-light conditions. Spiders use their secondary eyes for sensing motion, while their principal eyes allow shape and object recognition. In contrast to insect vision, a visually-based spider´s brain is almost completely devoted to vision, as it receives only the optic nerves and consists of only the optic ganglia and some association centers. The brain is apparently able to recognize object motion, but even more to also classify the counterpart into a potential mate, rival or prey by seeing legs (lines) at a particular angle to the body. Such stimulus will result in a spider displaying either courtship or threatening signs respectively.
A Spider´s eyes
[edit | edit source]Although spider eyes may be described as “camera eyes”, they are very different in their details from the “camera eyes” of mammals or any other animals. In order to fit a high-resolution eye into such a small body, neither an insect´s compound eyes nor spherical eyes, as we humans have them, would solve the problem. The ocelli found in spiders are the optically better solution, as their resolution is not limited by refractive effects at the lens which would be the case with compound eyes. When replacing the eye of a spider by a compound eye of the same resolving power, it would simply not fit into the spider´s prosoma. By using ocelli, the spatial acuity of some spiders is more similar to that of a mammal than to that of an insect, with a huge size difference and only a few thousand photocells, e.g. in a jumping spider´s eye, as compared to more than 150 million photocells in the human retina.
Principal eyes
[edit | edit source]
The anterior median eyes (AME), which are present in most spider species, are also called the principal eyes. Details about the principal eye´s structure and its components are illustrated in the figure below and are explained in the following by going through the AME of the jumping spider Portia (family Salticidae), which is famous for its high-spatial-acuity eyes and vision-guided behavior despite its very small body size of 4.5-9.5 mm.
When a light beam enters the principal eye it firstly passes a large corneal lens. This lens features a long focal length enabling it to magnify even distant objects. The combined field of view of the two principal eyes´ corneal lenses would cover about 90° in front of the salticid spider, however a retina with the desired acuity would be too large to fit inside a spider´s eye. The surprising solution is a small, elongated retina, which lies behind a long, narrow tube and a second lens (a concave pit) at its end. Such combination of a corneal lens (with a long focal length) and a long eye tube (magnifying the image from the corneal lens) resembles a telephoto system, making the pair of principal eyes similar to a pair of binoculars.
The salticid spider captures light beams successively on four retina layers of receptors, which lie behind each other (in contrast, the human retina is arranged in only one plane). This structure allows not only a larger number of photoreceptors in a confined area but also enables color vision, as the light is split into different colours (chromatic aberration) by the lens system. Different wavelengths of light thus come into focus at different distances, which correspond to the positions of the retina´s layers. While salticids discern green (layer 1 – ~580 nm, layer 2 – ~520-540 nm), blue (layer 3 – ~480-500 nm) and ultraviolet (layer 4 – ~360 nm) using their principal eyes, it is only the two rearmost layers (layers 1 and 2) which allow shape and form detection due to their close receptor spacing.
As in human eyes, there is a central region in layer 1 called the “fovea”, where the inter-receptor spacing was measured to about 1 μm. This was found to be optimal, as the telephoto optical system provides images precise enough to be sampled in this resolution, but any closer spacing would reduce the retina´s sampling quality due to quantum-level interference between adjacent receptors. Equipped with such eyes, Portia exceeds any insect by far when it comes to visual acuity: While the dragonfly Sympetrum striolatus has the highest acuity known for insects (0.4°), the acuity of Portia is ten times higher (0.04°) with much smaller eyes. The human eye with 0.007° acuity is only five times better than Portia´s. With such visual precision, Portia would be technically able to discriminate two objects which are 0.12 mm apart from a distance of 200 mm. The spatial acuity of other salticid eyes is usually not far behind that of Portia.[2][3][4]
Principal eye retina movements
[edit | edit source]Such spectacular visual abilities come at a price within small animals as the jumping spiders: The retina in each of Portia´s principal eyes has only 2-5° field of view, while its fovea even captures only 0.6° field of view. This results from the principal retina having elongated boomerang-like shapes which span about 20° vertically and only 1° horizontally, corresponding to about six receptor rows. This severe limitation is compensated by sweeping the eye tube over the whole image of the scene using eye muscles, of which jumping spiders have six. These are attached to the outside of the principal eye tube and allow the same three degrees of freedom – horizontal, vertical, rotation – as in human eyes. Principal retinae can move by as much as 50° horizontally and vertically and rotate about the optical axis (torsion) by a similar amount.
Spiders making sophisticated use of visual cues move their principal eyes´ retinae either spontaneously, in “saccades” fixating the fovea on a moving visual target (“tracking”), or by “scanning”, which serves presumably for pattern recognition. It seems today, that spiders scan a scene sequentially by moving the eye-tube in complex patterns, allowing it to process high amounts of visual information despite their very limited brain capacities.
The spontaneous retinal movements, so-called “microsaccades”, are a mechanism thought to prevent the photoreceptor cells of the anterior-median eyes from adapting to a motionless visual stimulus. Cupiennius spiders, which feature 4 eye muscles - two dorsal and two ventral ones – continuously perform such microsaccades of 2° to 4° in the dorso-median direction, lasting about 80 ms (when fixed to a holder). The 2-4° of microsaccadic movements match closely to Cupiennius´ angle of about 3° between the receptor cells, supporting the idea of its function preventing adaption. In contrast, retinal movements elicited by mechanical stimulation (directing an air puff onto the tarsus of the second walking leg) can be considerably larger than the spontaneous retinal movements, with deflections up to 15°. Such stimulus increases eye muscle activity from being spontaneously active at 12 ± 1 Hz at the resting level to 80 Hz with the air puff stimulation applied. Active retinal movement of the two principal eyes is however never activated simultaneously during such experiments and no correlation exists between the two eyes regarding their direction either. These two mechanisms, spontaneous microsaccades as well as active “peering” by active retinal movement, seemingly allow spiders to follow and analyze stationary visual targets efficiently using only their principal eyes without reinforcing the saccadic movements by body movements.
However, there is another factor influencing visual capacities of a spider´s eye, which is the problem of keeping objects at different distances in focus. In human eyes, this is solved by accommodation, i.e. changing the shape of the lens, but salticids take a different approach: the receptors in layer 1 of their retina are arranged on a “staircase” at different distances from the lens. Thus, the image of any object, whether a few centimeters or some meters in front of the eye, will be in focus on some part of the layer-1 staircase. Additionally, the salticid can swing the eye tubes side to side without moving the corneal lenses and will thus sweep the staircase of each retina across the image of the corneal lense, sequentially obtaining a sharp image of the object.
The resulting visual performance is impressive: Jumping spiders such as Portia focus accurately on an object at distances between 2 centimeters to infinity, being able to see up to about 75 centimeters in practice. The time needed to recognize objects is however relatively long (seemingly in the range of 10-20 s) because of the complex scanning process needed to capture high-quality images from such tiny eyes. Due to this limitation, it is very difficult for spiders such as Portia to identify much larger predators fast enough because of the predator´s size, making the small spider an easy prey for birds, frogs and other predators.[5][6]
Blurry vision for distance estimation
[edit | edit source]An unexpected finding recently surprised researchers, when it was shown that jumping spiders use a technique called blurry vision to estimate their distance to previously recognized prey before taking a jump. Where humans achieve depth perception using binocular vision and other animals do so by moving their heads around or measuring ultrasound responses, jumping spiders perform this task within their principal eyes. As in other jumping spider species, the principal eyes of Hasarius adansoni feature four retinal layers with the two bottom ones featuring photocells responding to green impulses. However, green light will only ever focus sharply on the bottom one, layer 1, due to its distance from the inner lens. Layer 2 would receive focused blue light, however these photoreceptor cells are not sensitive to blue and receive a fuzzy green image instead. Interestingly, the amount of blur depends on the distance of an object from the spider´s eye – the closer it is, the more out of focus it will appear on the second retina layer. At the same time, the first retina layer 1 always receives a sharp image due to its staircase structure. Jumping spiders are thus able to estimate depth using a single unmoving eye by comparing the images of the two bottom retina layers. This was confirmed by letting spiders jump at prey in an arena flooded with green light versus red light of equal brightness. Without the ability to use the green retina layers, jumping spiders would repeatedly fail to judge distance accurately and miss their jump.
Secondary eyes
[edit | edit source]
In contrast to the principal eyes responsible for object analysis and discrimination, a spider´s secondary eyes act as motion detectors and therefore do not feature eye muscles to analyze a scene more extensively. Depending on their arrangement on the spider´s carapace, secondary eyes enable the animal to have panoramic vision detecting moving objects almost 360° around its body. The anterior and posterior lateral eyes (i.e. secondary eyes) only feature a single type of visual cells with a maximum spectral sensitivity for green colored light of ~535-540 nm wavelength. The number and arrangement of secondary eyes differs significantly between or even within different spider families, as does their structure: Large secondary eyes can contain several thousand rhabdomeres (the light-sensitive parts of the retina) and support hunters or nocturnal spiders with their high sensitivity to light, while small secondary eyes contain at most a few hundred rhabdomeres and only providing basic movement detection. Differently from the principal eyes which are everted (the rhabdomeres point towards the light), the secondary eyes of a spider are inverted, i.e. their rhabdomeres point away from the light, as is the case for vertebrates like the human eye. Spatial resolution of the secondary eyes e.g. in the extensively studied Cupiennius salei is greatest in horizontal direction, enabling the spider to analyse horizontal movements well even with the secondary eyes, while vertical movement may not be especially important when living in a “flat world”.
The reaction time of jumping spiders´ lateral eyes is comparably slow and amounts to 80-120 ms, measured with a 3°-sized (inter-receptor angle) square stimulus travelling past the animal´s eyes. The minimum stimulus travel distances, until the spider reacts, are 0.1° at a stimulus velocity of 1°/s, 1° at 9°/s and 2.5° at 27°/s. This means that a jumping spider´s visual system detects motion even if an object is travelling only a tenth of the secondary eyes´ inter-receptor angle at slow speed. If the stimulus gets even smaller to a size of only 0.5°, responds occur only after long delays, indicating that they lie at the spiders´ limit of perceivable motion.
Secondary eyes of (night-active) spiders usually feature a tapetum behind the rhabdomeres, which is a layer of crystals reflecting light back to the receptors to increase visual sensitivity. This allows night-hunting spiders to have eyes with an aperture as large as f/0.58 enabling them to capture visual information even in ultra-low-light conditions. Secondary eyes containing a tapetum thus easily reveal a spider´s location at night when illuminated e.g. by a flashlight.[7][8]
Central nervous system and visual processing in the brain
[edit | edit source]As anywhere in neuroscience, we still know very little about a spider´s central nervous system (CNS), especially regarding its functioning in visually controlled behavior. Of all the spiders, the CNS of Cupiennius has been studied most extensively, focusing mainly on the CNS structure. As of today, only little is known about electrophysiological properties of central neurons in Cupiennius, and even less about other spiders in this regard.
The structure of a spider´s nervous system is closely related to its body´s subdivisions, but instead of being spread all over the body, the nervous tissue is enormously concentrated and centralized. The CNS is made up of two paired, rather simple nerve cell clusters (ganglia), which are connected to the spider´s muscles and sensory systems by nerves. The brain is formed by fusion of these ganglia in the head segments ahead of and behind the mouth and fills the prosoma largely with nervous tissue, while no ganglia exist in the abdomen. Looking at the spider´s brain, it receives direct inputs from only one sensory system, the eyes - unlike any insects and crustaceans. The eight optic nerves enter the brain from the front and their signals are processed in two optic lobes in the anterior region of the brain. When a spider´s behavior is especially dependent on vision, as in the case of the jumping spider, the optic ganglia contribute up to 31% of the brain´s volume, indicating the brain to be almost completely devoted to vision. This score still amounts to 20% for Cupiennius, whereas other spiders like Nephila and Ephebopus come in at only 2%.
The distinction between principal and secondary eyes persists in the brain. Both types of eyes have their own visual pathway with two separate neuropil regions fulfilling distinct tasks. Thus spiders evidently process the visual information provided by their two eye types in parallel, with the secondary eyes being specialized for detecting horizontal movement of objects and the principal eyes being used for the detection of shape and texture.
Two visual systems in one brain
[edit | edit source]While principal and secondary eyesight seems to be distinct in spiders´ brains, surprising inter-relations between both visual systems in the brain are known as well. In visual experiments principal eye muscle activity of Cupiennius was measured while covering either its principal or secondary eyes. When stimulating the animals in a white arena with short sequences of moving black bars, the principal eyes moved involuntarily whenever a secondary eye detected motion within its visual field. This activity increase of the principal eye muscles, compared to no stimulation presented, would not change when covering the principal eyes with black paint, but would stop with the secondary eyes masked. Thus it is now clear, that only the input received from secondary eyes controls principal eye muscle activity. Also, a spider´s principal eyes do not seem to be involved in motion detection, which is only the secondary eyes´ responsibility.
Other experiments using dual-channel telemetric registration of the eye muscle activities of Cupiennius have shown that the spider actively peers into the walking direction: The ipsilateral retina of the principal eyes was measured to shift with respect to the walking direction before, during and after a turn, while the contralateral retina remained in its resting position. This happened independently from the actual light conditions, suggesting a “voluntary” peering initiated by the spider´s brain.
Pattern recognition using principal eyes
[edit | edit source]
Recognition of shape and form by jumping spiders is believed to be accomplished through a scanning process of the visual field, which consists of a complex set of rotations (torsional movements) and translations of the anterior-median eyes´ retinae. As described in the section “Principal eye retina movements”, a spider´s retinae are narrow and shaped like boomerangs, which can be matched with straight features by sweeping over the visual scene. When investigating a novel target, the eyes scan it in a stereotyped way: By moving slowly from side to side at speeds of 3-10° per second and rotating through ± 25°, horizontal and torsional retina movement allows the detection of differently positioned and rotated lines. This method can be understood as template matching where the template has elongated shape and produces a strong neural response whenever the retina matches a straight feature in the scene. This identifies a straight line with little or no further processing necessary.
A computer vision algorithm for straight line detection as an optimization problem (da Costa, da F. Costa) was inspired by the jumping spider´s visual system and uses the same approach of scanning a scene sequentially using template matching. While the well-known Hough Transform allows robust detection of straight visual features in an image, its efficiency is limited due to the necessity to calculate a good part or even the whole parameter space while searching for lines. In contrast the alternative approach used in salticid visual systems suggests searching the visual space by using a linear window, which allows adaptive searching schemes during the straight line search process without the need to systematically calculate the parameter space. Also, solving the straight line detection in such a way allows to understand it as an optimization problem, which makes efficient processing by computers possible. While it is necessary to find appropriate parameters controlling the annealing-based scanning experimentally, the approach taking a jumping spider´s path of straight line detection was proven to be very effective, especially with properly set parameters.[9]
Visually-guided behavior
[edit | edit source]Discernment of visual targets
[edit | edit source]
The ability of discerning between slightly different visual targets has been shown for Cupiennius salei, although this species relies mainly on its mechanosensory systems during prey catching or mating behavior. When presenting two targets at a distance of 2 m to the spider, its walking path depends on their visual appearance: Having to choose between two identical targets such as vertical bars, Cupiennius shows no preference. However the animal strongly prefers a vertical bar to a sloping bar or a V-shaped target.
The discrimination of different targets has been shown to be only possible with the principal eyes uncovered, while the spider is able to detect the targets using any of the eyes. This suggests that many spiders´ anterior-lateral (secondary) eyes are capable of much more than simply object movement detection. With all eyes covered, the spider exhibits totally undirected walking paths.
Placing Cupiennius in total darkness however results not only in undirected walks but also elicits a change of gait: Instead of using all eight legs the spider will only walk with six and employ the first legs as antennae, comparable to a blind person´s cane. In order to feel the surroundings the extended forelegs are moved up and down as well as sideways. This is specific to the first leg pair only, influenced solely by the visual input when the normal room light is switched to the invisible infrared light.
Vision-based decision making in jumping spiders
[edit | edit source]The behavior of jumping spiders after having detected movement with the eyes depends on three factors: the target´s size, speed and distance. If it has more than twice the spider´s size, the object is not approached and the spider tries to escape if it comes towards her. If the target has adequate size, its speed is visually analyzed using the secondary eyes. Fast moving targets with a speed of more than 4°/s are chased by jumping spiders, guided by her anterior-lateral eyes. Slower objects are carefully approached and analyzed with the anterior-median (i.e. principal) eyes to determine whether it is prey or another spider of the same species. This is seemingly achieved by applying the above described straight line detection, to find out whether a visual target features legs or not. While jumping spiders have shown to approach potential prey of appropriate characteristics as long as it moves, males are pickier in deciding whether their current counterpart might be a potential mate.
Potential mate detection
[edit | edit source]Experiments have shown that drawings of a central dot with leg-like appendages on the sides will result in courtship displays, suggesting that visual feature extraction is used by jumping spiders to detect the presence and orientation of linear structures in the target. Additionally, a spider´s behavior towards a considered conspecific spider depends on different factors such as sex and maturity of both involved spiders and whether it is mating time. Female wolf spiders, Schizocosa ocreata, even discern asymmetries in male secondary sexual characters when choosing their mate, possibly to avoid developmental instability in their offspring. Conspicuous tufts of bristles on a male´s forelegs, which are used for visual courtship signaling, appear to influence female mate choice and asymmetry of these body parts in consequence of leg loss and regeneration apparently reduces female receptivity to such male spiders.[10]
Secondary eye-guided hunting
[edit | edit source]A jumping spider´s stalking behavior when hunting insect prey is comparable to a cat stalking birds. If something moves within the visual field of the secondary eyes, they initiate a turn to bring the larger, forward-facing pair of principal eyes into position for classifying the object´s shape into mate, rival or prey. Even very small, low contrast dot stimuli moving at slow or fast speeds elicit such orientation behavior. Like Cupiennius, jumping spiders are also able to use their secondary eyes for more sophisticated tasks than just motion detection: Presenting visual prey cues to salticids with only visual information from the secondary eyes available and both primary eyes covered, results in the animal exhibiting complete hunting sequences. This suggests that the anterior lateral eyes of jumping spiders may be the most versatile components of their visual system. Besides detecting motion, the secondary eyes obviously also feature a spatial acuity which is good enough to direct complete visually-guided hunting sequences.
Prey “face recognition”
[edit | edit source]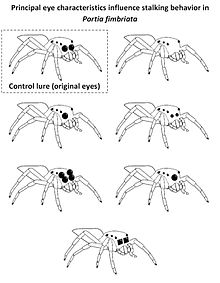
Visual cues also play an important role for jumping spiders (salticids) when discriminating between salticid and non-salticid prey using principal eyesight. To this end a salticid prey´s large principal eyes provide critical cues, to which the jumping spider Portia fimbriata reacts by exhibiting cryptic stalking tactics before attacking (walking very slowly with palps retracted and freezing when faced). This behavior is only used when identifying a prey as salticid. This was exploited in experiments presenting computer-rendered, realistic three-dimensional lures with modified principal eyes to Portia fimbriata. While intact virtual lures resulted in cryptic stalking, lures without or with smaller principal eyes than usual (as sketched in the figure on the right) elicited different behavior. Presenting virtual salticid prey with only one anterior-median eye or a regular lure with two enlarged secondary eyes elicited cryptic stalking behavior suggesting successful recognition of a salticid, while P. fimbriata froze less often when faced by a Cyclops-like lure (a single principal eye centered between the two secondary eyes). Lures with square-edged principal eyes were usually not classified as a salticid, indicating that the shape of the principal eyes´ edges are an important cue to identify fellow salticids.[11]
Jumping decisions from visual features
[edit | edit source]
Spiders in the genus Phidippus have been tested within a study for their willingness to cross inhospitable open space by placing visual targets on the other side of a gap. It was found that whether the spider takes the risk of crossing open ground or not is mainly dependent on factors like distance to target, relative target size compared to distance and the target´s color and shape. In independent test runs, the spider moved to tall, distant targets equally often as to short, close targets, with both objects appearing equally sized on the spider´s retina. When giving the choice of moving to either white or green grass-like targets, the spiders consistently chose the green target irrespective of its contrast with the background, thus proving their ability to use color discernment in hunting situations.[12]
Identifying microhabitat traits by visual cues
[edit | edit source]Presented with manipulated real plants and photos of plants, Psecas chapoda (a bromeliad-dwelling salticid spider) is able to detect a favorable microhabitat by visually analyzing architectural features of the host plant´s leaves and rosette. By using black-and-white photos, any potential influence of other cues, such as color and smell, on host plant selection by the spider could be excluded during a study, leaving only shape and form as discerning characteristics. Even when having to decide solely from photographs, Psecas chapoda consistently preferred rosette-shaped plants (Agavaceae) with narrow and long leaves over differently looking plants, which proves that some spider species are able to evaluate and distinguish physical structure of microhabitats only on the basis of shape from visual cues of plant traits.[13]
- ↑ F. G. Barth: A Spider´s World: Senses and Behavior. ISBN 978-3-642-07557-5, Springer-Verlag Berlin, Heidelberg. (2002)
- ↑ D. P. Harland, R. R. Jackson: 'Eight-legged cats' and how they see - a review of recent research on jumping spiders (Araneae: Salticidae). Department of Zoology, University of Canterbury (2000)
- ↑ A. Schmid: Different functions of different eye types in the spider Cupiennius salei. The Journal of Experimental Biology 201, 221–225 (1998)
- ↑ S. Yamashita, H. Tateda: Spectral Sensitivities of Jumping Spider Eyes. J. comp. Physiol. 105, 29-41 (1976)
- ↑ D. P. Harland, R. R. Jackson: Influence of cues from the anterior medial eyes of virtual prey on Portia fimbriata, an araneophagic jumping spider. The Journal of Experimental Biology 205, 1861–1868 (2002)
- ↑ A. Schmid, C. Trischler: Active sensing in a freely walking spider: Look where to go. Journal of Insect Physiology 57 p.494–500 (2011)
- ↑ D. B. Zurek, X. J. Nelson: Hyperacute motion detection by the lateral eyes of jumping spiders. Vision Research 66 p.26–30 (2012)
- ↑ D. B. Zurek, A. J. Taylor, C. S. Evans, X. J. Nelson: The role of the anterior lateral eyes in the vision-based behaviour of jumping spiders. The Journal of Experimental Biology 213, 2372-2378 (2010)
- ↑ F. M. G. da Costa, L. da F. Costa: Straight Line Detection as an Optimization Problem: An Approach Motivated by the Jumping Spider Visual System. In: Biologically Motivated Computer Vision, First IEEE International Workshop, BMVC 2000, Seoul, Korea (2000)
- ↑ G.W. Uetz, E. I. Smith: Asymmetry in a visual signaling character and sexual selection in a wolf spider. Behav Ecol Sociobiol (1999) 45: 87–93
- ↑ D. P. Harland, R. R. Jackson: Influence of cues from the anterior medial eyes of virtual prey on Portia fimbriata, an araneophagic jumping spider. The Journal of Experimental Biology 205, 1861–1868 (2002)
- ↑ R. R. Jackson, D. P. Harland: One small leap for the jumping spider but a giant step for vision science. THE JOURNAL OF EXPERIMENTAL BIOLOGY, JEB Classics p.2129-2132
- ↑ P. M. de Omena, and G. Q. Romero: Using visual cues of microhabitat traits to find home: the case study of a bromeliad-living jumping spider (Salticidae). Behavioral Ecology 21:690–695 (2010)