Structural Biochemistry/Cell Signaling Pathways/Apoptosis
Background
[edit | edit source]Apoptosis or programmed cell death is an important biological process. It helps body to get rid of cells that are superfluous or potentially harmful. In addition, it helps to shape our feature by removing cells that are no longer needed. When a cell undergoes apoptosis, its DNA is cleaved into fragment by an “apoptosis-specific DNA endonuclease” known as DNase. “Eventually, cell is dismantled into small pieces called apoptotic bodies” which will be engulfed by the specialized cells nearby (Becker et al. 419-420). The process is carried out by chromatin condensation and fragmentation; it is executed by the formation of multi-protein complexes that involve the binding of extracellular death ligands to death receptors. The complexes generate and activate initiator caspases, which then trigger and cleave effector caspases that then target and focus on specific cellular substrates for the process of proteolysis. Caspases is the key enzyme involved in apoptosis and it is catalytically active only during this time.They are directly inhibited by members of the IAP family. The inactivated form of this enzyme is called procaspases which is activated by a different caspases through proteolytic cleavage. Once it is turned on, caspases will cleave the inhibitory protein on DNase to induce cell death (420).
There are two major types of signals that can trigger apoptosis in human, death signals and withdrawal of survival signals (420). In the death signal pathway, a cell that is infected with virus or bacterial will accumulate CD9 or similar proteins on its surface. These proteins are called death signals that will attract procaspases to cell’s surface. Once procaspases is turned on, it will activate more procaspases to initiate apoptosis. In the survival factor pathway, mitochondria play an important role in programming cell death. Proteins called anti-apoptotic proteins reside on the outer membrane of mitochondria. The function of those proteins is to prevent apoptosis as long as the cells are exposed to survival factors (421). Another type of proteins called pro-apoptotic proteins which can promote apoptosis exist in balance with anti-apoptotic proteins. Once the survival factor is withdrawn from cell, this balance will be broken, causing equilibrium to shift more toward pro-apoptotic proteins. As a result, there is an increasing in chance for cell to undergo apoptosis (421).
Apoptosis in Neurons
[edit | edit source]All neurons have suicide genes, and these genes are influenced by brain chemistry and activation patterns. Fetal development is a very competitive process because at this stage, the nervous system massively overproduces cells (produces more than 50% the amount that survive). As this huge amount of cells compete for connections, those who are not able to make a connection die off because their suicide genes are activated. This competition for connections consists of axons fighting for space on the Post-Synaptic cell: axons begin by branching widely and connecting to many sites, and the few that are strengthened through positive feedback (in this case, recurring signals between the axon and the post-synaptic cell) are the ones that are maintained. As a result, each cell makes fewer and more selective connections as prenatal development progresses. On the other hand, the others who lose the competition and fail to receive positive feedback go through apoptosis (suicide genes are activated)
Apoptosis and necrosis differ in the sense that necrosis results in messy byproducts from injury or toxins, which can cause contamination or inflammation. This sort of cell death is injurious and deleterious, whereas apoptosis is an essential and neat process where cell death does not leave byproducts or unnecessary material behind.
Apoptosis in Caenorhabditis elegans
[edit | edit source]Caenorhabditis elegans, or C. elegans, are a type of nematode where research is undergone to better understand apoptosis in multicellular organisms. Research can be done with the C. elegans by the fact that throughout the C. elegans' development, the cell numbers are regulated and controlled in about all adult worms. Therefore, all the adult worms contain about the same number of cells in each mature organ. The executioner caspase in C. elegans is CED-3, which in turn triggers the apoptosis.
The apoptosis in C. elegans rank similar to apoptosis in higher organisms because the CED-3 caspase in C. elegans contain homologs to the caspases in mammals, e.g. caspase -3 and caspase-8. CED-3 is synthesized as an inactive zymogen; dimerization and autoproteolysis generate the active components (the large and small subunits) from the N-terminal prodomain. The oligomerized CED-4 bring together and active the before inactive CED-3 monomers, a parallel process of mammalian caspase-9 activation.
A way for C. elegans regulates the activation of CED-3 and apoptosis is recently found to be CSP-3; a cytoplasmic protein that mimics the CED-3 small subunit, binding to and sequestering the CED-3 zymogen. Before, CSP-3 was found to be a negative regulator of apoptosis in cells that should survive, but it did not block the appropriate induction of apoptosis in cells that should die. Therefore, CSP-3 was considered a caspase-like gene and scientists were still clueless on the true origin of regulation. Recent research shows on the other hand that the CSP-3 is able to bind the CED-3 zymogen in vitro and in vivo, and that CSP-3 is a modulator of CED-3 activation instead of a true caspase inhibitor. This demonstrates that CSP-3 does prevent inappropriate CED-3 dimerization and autoactivation. On a side note, CED-4 is a gene that can override the CSP-3 and its effects on CED-3 and apoptosis.
Cellular Apoptosis and Mitochondria
[edit | edit source]ATP production is not the only invaluable skill mitochondria possess. They are a vital component of the cell’s cellular transduction network that is capable of triggering apoptosis, or programmed cell death. The primary proteinic signal that initiates this process is cytochrome-c, an electron transporting membrane protein, which releases chemical messengers that activate caspases and lead the cell to following an apoptotic program that shuts it down. So, even though mitochondria are constantly providing the cell with energy through maintaining an ionic gradient that converts ADP into ATP by way of ATP Synthase, the organelle is also capable of inflicting cellular suicide by over-expressing the same membrane proteins that perform electron transport to maintain that ionic gradient. To prevent unintentional initiation of apoptosis, the organelle relies on the expression of bcl-2 to counteract the effects of cytochrome-c by preventing its translocation from the mitochondria.
Additional Forms of Cell Death
[edit | edit source]Autophagy
[edit | edit source]Autophagy is a process described as a pro-survival pathway that is considered essential for cellular homeostasis and stress responses. It accompanies cell death and is capable of leading to cell death under certain circumstances. This process is carried out by the Atg genes; during this period, intracellular contents are consumed by either double or multi-membrane vesicles called autophagosomes. The suppression of the mammalian target of rapamycin kinase is necessary for the progression of autophagy because the rapamycin kinases release its inhibitory effects on the ULK1 kinase complex. Autophagy is in charge of the turnover of long-lived proteins, the elimination of misfolded proteins and some damaged organelles, and also the recycling of cellular building blocks after nutrient depravation. Therefore autophagy is an essential pro-survival pathway in cellular homeostasis and also stress because it can actually carry out cell death.
Necrosis
[edit | edit source]Necrosis was originally categorized as accidental cell death, but due to recent research, it is now recognized as a genetically controlled event. This process involves various characteristics such as: cell swelling, organelle dysfunction, and cell lysis. Necrosis does not have precise mechanisms identified, but a few regulators such as c-Jun N-terminal kinase, apoptosis inducing factor, death-associated protein kinase, and reactive oxygen species have been identified and associated with necrosis. A sub-category of necrosis is known as necroptosis, which is triggered by death receptors in cells that are apoptosis incompetent.
Picture of Apoptosis/Autophagy/Necrosis Click Link Below
- REDIRECT [[1]]
Regulators of Necrosis
[edit | edit source]Apoptosis-Inducing Factor (AIF)
[edit | edit source]Although AIF is one of many regulators for programmed cell death (PCD), it has other unique functions that allows itself to be differentiated from other apoptosis factors shown in the picture. It was at first discovered as a caspase-independent death effector but later studies have shown that it is directly related to programmed cell death pathways that particular types of cells take. AIF performs its deadly function when it is signaled to be released from mitochondria and is translocated initially to the cytosol then to the nucleus of the targeted cell. At the nucleus, it can induce apoptosis related features such as DNA fragmentation and chromatin condensation. AIF’s effect is entirely dependent on its intrinsic DNA binding abilities as well as the cell type of the target cell because AIF has later been discovered to have crucial mitochondrial roles in healthy cells that are not tagged to have programmed cell death. AIF has a role in DNA binding as well. These studies lead to many questions as to AIF’s essential role in the body and if proper functions can be carried out in its absence.
Structure and Function
[edit | edit source]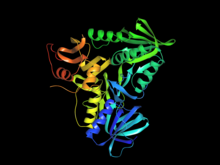
Mitochondrial AIF proteins, as mentioned above, have a lethal purpose to facilitate programmed cell death. It does so upon mitochondrial outer membrane permeabilization (MOMP), after which it will be released into the cytosol and eventually to the nucleus where it condenses chromatin and degrades DNA fragments to carry out PCD. Primary AIF transcripts go through very tissue-specific splicing and create two exons: exon 2a and 2b. The two exons allow for production of two splice variants and create two isoforms that show a small and limited difference found in the inner membrane-sorting signal (IMSS). The N-terminal mitochondrial localization signal (MLS) is absent in these variants, but the C-terminal domain remains unaffected. The C-terminal domain is where the pro-apoptotic segment is located, and upon transfection (or more commonly termed transformation) the variant genes (AIFsh) are expressed in the nucleus and activate apoptosis. There are variations of the AIFsh that include isoforms that do not have the C-terminal domain with the apoptotic features or another form that lacks the mitochondrial localization signal and essentially is rendered to have similar functions as the AIFsh lacking the C-terminal domain.
X-ray crystallography has provided pictures of the AIF structure that has led to another way that AIF carries out its function. The structure shows that AIF possesses positively charged amino acids that are distributed throughout its surface; this distribution of positive charge is very similar to that of DNA-binding histones. This discovery explains and is consistent with the observation of AIF’s intrinsic DNA-binding capabilities and how effective it is as an apoptosis regulator. A few of the positive residues are required for AIF-nucleic acid binding and for inducing nuclear apoptosis due to the overexpression of AIF. The recombinant AIF genes induces DNA condensation through direct, sequence-independent interactions with the DNA, whether it be single- or double-stranded. The DNA condensation can be amplified by adding reduced pyridine nucleotides, NADPH, or NADH.
Evolutionary Family
[edit | edit source]Other regulators of apoptosis come from the same larger mitochondrial flavoprotein family that AIF belongs too. They have common structural and functional features in eukaryotic versions of the protein. But AIF has a slightly different biosynthesis and finalizing processing than the other members of the family. The AIF gene consists of over 16 exons and is located on the human chromosome X. AIF1, variant AIF gene with the C-terminal domain, is the most common and abundant transcript. It is translated in the cytoplasm and sent to the mitochondria of healthy cells. Its N-terminal part is required for the import of the AIF protein through the membrane (outer and inner) of the mitochondria. This N-terminal part is encoded by the first exon of 35 amino acids that make up the gene. As the fully processed AIF protein is inserted in the inner membrane, it matures and folds into its quaternary structure using its co-factor flavin adenine dinucleotide (FAD). Because of AIF’s dependence on its cofactor, it has similar folds as the bacterial NAD and contains two FAD-binding segments. These features were conserved through the evolution of these different proteins.
AIF Regulation
[edit | edit source]AIF can be both triggered and inhibited (or regulated) by other metabolic processes or proteins. AIF expression has been correlated to the sensitivity of anti-cancer drugs. Its reduced expression has also been seen when hepatocyte growth factors have been added to the cell, which increases cisplatin resistance. Increased cisplatin sensitivity is often seen with increased expression of AIF. In mouse embryonic fibroblasts that lack FAK (focal adhesion kinase), it is observed that there’s a smaller amount of HGF which exhibit the aforementioned correlations. Most of these studies are only clinical and have been performed on only mice.
The amount of AIF proteins are also regulated after translation. Its concentration is positively regulated by scythe, also referred to as BAT3. BAT3 is a protein used in the regulation of cell death and cell proliferation. Scythe has been known to prolong the half-life of AIF, and when its levels are low it promotes AIF breakdown. Other inhibitors of apoptosis have been known to trigger the degradation of AIF. However, these occurrences have little to no effect on the AIF proteins found in the inner mitochondrial membrane which have yet to be modified to be released into the cytosol.
AIF and the Mitochondria
[edit | edit source]Lack of AIF expression leads to a defect in the mitochondrial respiratory chain, especially in complex I. This observation again was only experimented on mice. A significant detection of malfunction in the respiratory chain was located in various tissues such as the cerebellum, cortex, retina, and skeletal muscles. Malfunction, however, could not be detected on tissues of the heart, liver, and testis of male mice. Researchers are still perplexed at how tissue-specific the defect is. Analyses do show that the deficiency in complex I activity in mouse and human cells that lack AIF is due to a decreased expression of various other nuclear-encoded protein subunits found in the respiratory chain. There are 46 total protein subunits that make up complex I, and 39 of which are nuclear-encoded to be translated int the cytosol and taken into the mitochondria. It has been shown that overexpression of a mitochondrion-localized AIF fixes the malfunctions in complex I subunits that are caused by endogenous AIF proteins. Although this evidence shows valuable insight, mechanisms that lead to inefficiencies of complex I are still up to greater debate and research. Results of various recent experiments have shown inconsistent observations that may or may not link AIF directly to the complex I.
Many hypotheses have been made about AIF’s relation to complex I:
- AIF levels affect the high level of complex I proteins through post-transcriptional mechanisms
- AIF is an integral part of the mitochondrial respiratory chain complexes, not just related to complex I
- Physical interactions of the AIF proteins with the respiratory chain subunits could affect their assembly or stability
- AIF could be a part of the maintenance of the active complex I due to its redox-regulated activity which is inherent because of AIF’s outer and reactive positive charge distribution
AIF’s Role in Development/Survival
[edit | edit source]Although AIF has been known for years of its function to induce apoptosis, it also has essential functions in cell survival because of its role in the metabolism of cells and in the mitochondria. As of recently, studies of AIF’s role in survival have been exclusively done on mice. Having low continuous amounts of AIF throughout an aging adult’s lifetime can have dire health consequences. An approximate 80% decrease in AIF gene expression in mice has shown continual neurodegeneration that affects the mice’s various brain regions. When a complete absence of AIF proteins in the mice was observed, apoptosis still occurred in its usual fashion. This leads to the conclusion that AIF in terms of apoptotic function is dispensable, especially during embryonic neural tube closure.
Rather than using AIF, the triggering of programmed cell death was done by defective activity of the mitochondria’s respiratory chain complex I. Lack of AIF expression in the brain is directly linked to the inefficiencies of the mitochondrial respiratory chain. Because of its malfunction, the midbrain and cerebellum of the mice at early stages of brain development were observed to have defects such a cerebellar hypoplasia. The Purkinje precursor cells of these embryos undergo apoptosis even in the absence of AIF as well. Granule cells also show defective cell cycle transitions (particularly from G1 to S steps). AIF in skeletal muscle and the myocardium causes organ-specific complex I malfunction. Although these mice look normal for the first 2 months since birth, they develop muscle atrophy, dilated cardiomyopathy, and have a dramatic reduced body weight. Decreased expression of AIF leads to oxidative phosphorylation complications that affect the body’s entire metabolism. Mice with absence of AIF in either their muscles or life (called AIF knockout) display higher glucose tolerance, increased sensitivity to insulin, and overall reduced fat mass. These observations imply that no AIF expression in one organ leads to it being fully resistant to obesity and diabetes even when put on a diet high of lipids.
References
[edit | edit source]- Becker, Wayne M, et al. The World of the Cell. 7th ed. New York: Pearson/Benjamin Cummings, 2009. Print
- Klein, Stephen B. "Biological Psychology". New York: Worth Publishers, 2006. Print
- Brady, Graham F, Brady G.F., Duckett C.S. "A caspase homolog keeps CED-3 in check". (2009) Trends in Biochemical Sciences, 34 (3), pp. 104-107.
- Schatz, Gottfried. "The Magic Garden." Annual Review of Biochemistry 76.1 (2007): 673-78. Print.
- Jie Yang, Xuesong Liu*, et al. "Prevention of Apoptosis by Bcl-2: Release of Cytochrome c from Mitochondria Blocked" Science 21 February 1997: Vol. 275 Print
- "Developmental Apoptosis in : C. Elegans: : a Complex CEDnario : Abstract : Nature Reviews Molecular Cell Biology." Nature Publishing Group : Science Journals, Jobs, and Information. Web. 30 Nov. 2011. <http://www.nature.com/nrm/journal/v7/n2/abs/nrm1836.html>.
- "Neurotoxicity: Mitochondria." Macalester College: Private Liberal Arts College. Web. 30 Nov. 2011. <http://www.macalester.edu/psychology/whathap/UBNRP/meth08/neurotoxicity/methmito.html>.
- "Mitochondria and Apoptosis." St Georges University of London - Home. Web. 30 Nov. 2011. <http://www.sgul.ac.uk/depts/immunology/~dash/apoptosis/mito.htm>.
- Ber, Yaara, Rubinstein, Assaf, KimchI, Adi. "Systems biology analysis of programmed death". Elsevier Ltd (2010). Print
- Life with or without AIF. Hangen E, Blomgren K, Bénit P, Kroemer G, Modjtahedi N. Trends Biochem Sci. 2010 May. Web. Home. 6 Dec. 2012. <http://www.sciencedirect.com/science/article/pii/S0968000410000022#>