9-1 Chemistry/Printable version
This is the print version of 9-1 Chemistry You won't see this message or any elements not part of the book's content when you print or preview this page. |
The current, editable version of this book is available in Wikibooks, the open-content textbooks collection, at
https://en.wikibooks.org/wiki/9-1_Chemistry
The periodic table
The periodic table
[edit | edit source]The elements in the periodic table are arranged in order of their atomic, proton, number. Each column, known as a group, contains elements with similar properties as they all have the same number of electrons in their outer shell.
Metals and non-metals
[edit | edit source]Metals are elements which react to form positive ions whilst non-metals react to form negative ions. The majority of elements are metals and are found on the left side of the periodic table whilst the few non-metals are found at the top-right.

Development of the periodic table
[edit | edit source]Döbereiner's triads
[edit | edit source]A German scientist called Johann Döbereiner put forward his law of triads in 1817. Each of Döbereiner's triads was a group of three elements. The appearance and reactions of the elements in a triad were similar to each other. See the table below:
symbol | A (atomic mass) |
---|---|
Li | 7 |
Na | 23 |
K | 39 |
Döbereiner discovered that the relative atomic mass of the middle element in each triad was close to the average of the relative atomic masses of the other two elements. However, his law worked only for few elements, and hence soon fell out of favour. [1]
Newlands' Law of Octaves
[edit | edit source]An English scientist called John Newlands put forward his law of octaves in 1864. He arranged all the elements known at the time into a table in order of relative atomic mass. When he did this, he found that each element was similar to the element eight places further on. For example, starting at Li, Be is the second element, B is the third and Na is the eighth element. Newlands' table showed a repeating or periodic pattern of properties, but it had problems. For example, he put iron in the same group as oxygen and sulphur, which are two non-metals. As a result, his table was not accepted by other scientists. [2]
Mendeleev's periodic table
[edit | edit source]In 1869, just five years after John Newlands put forward his law of octaves, a Russian chemist called Dmitri Mendeleev published a periodic table. Mendeleev also arranged the elements known at the time in order of relative atomic mass, but he also realized that that the physical and chemical properties of elements were related to their atomic mass in a 'periodic' way, and arranged them so that groups of elements with similar properties fell into vertical columns in his table. [3]

In the table above, you can see that some elements have been marked with a dash (-). Those elements were not discovered at that time, so he marked (predicted) them as eka-<a compound with similar specifications>. For example, gallium and eka-aluminium have similar properties, as you can see in the table below:
Eka-Aluminum (Ea) | Gallium (Ga) | |
Atomic mass | 68 amu | 69.9 amu |
Melting point | Low | 30.15°C |
Density | 5.9 g/cm 3 | 5.94 g/cm 3 |
Formula of oxide | Ea 2 O 3 | Ga 2 O 3 |
The element gallium was discovered four years after the publication of Mendeleev’s table, and its properties matched up remarkably well with eka-aluminum, fitting into the table exactly where he had predicted. This was also the case with the element that followed gallium, which was named eventually named germanium.
Mendeleev’s periodic table gained wide acceptance with the scientific community and earned him credit as the discoverer of the periodic law. Element number 101, synthesized in 1955, is named mendelevium after the founder of the periodic table.[4]
Group 0: Noble gases
[edit | edit source]The elements on the far right of the periodic table are known as the noble gases, Group 0. They are very unreactive as they have a full outer shell, giving them a naturally stable arrangement of electrons (they do not have to react to form a stable arrangement). So we say they are inert - extremely unreactive and do not take part in chemical reactions and they exist as single atoms (monatomic).
The boiling points of the noble gases increase with increasing relative atomic mass, so the further down in the group you go, the higher the boiling point. This is as a result of greater intermolecular forces as atoms get bigger resulting in more energy being needed to break the bonds and change state. However, compared to metals they all have very low boiling and melting points and usually exist as gases at room temperature.
Group 1: Alkali Metals
[edit | edit source]The elements on the far left of the periodic table are known as the Alkali Metals or Group 1 (since they all have 1 electron in their outer shell). In order to form a stable arrangement, they need to lose 1 electron, resulting in them becoming a positive ion (1+). All alkali metals are soft (as they can be easily cut with a knife) and have relatively low melting and boiling points.

Alkali metals get there name from their reaction with water:
Alkali metals react with water to produce an alkali metal hydroxide and hydrogen. The hydroxide is an alkali, with a pH greater than 7, which is able to dissolve in water, turning a universal indicator blue or purple.
The reactivity of alkali metals increases going down the group since there is a higher shielding effect created by more filled electron shells, meaning that electrons can be lost more easily. This means that if francium were to react with water, there would be an explosion.
Alkali metals react with oxygen to produce an alkali metal oxide. This explains why when you cut an alkali metal the shiny surface quickly dulls as an oxide layer forms, having reacted with oxygen. It also explains why alkali metals burn vigorously when you place them in a jar filled with oxygen. The oxide will form as a white smoke.
Group 1 metals react with chlorine to produce (chlor)ides, a compound containing chlorine and another element.
Group 7: Halogens
[edit | edit source]Halogens are the Group 7 elements in the periodic table and have 7 electrons in their outer shell. The halogens are non-metals and consist of diatomic (a pair of halogen atoms bonded together) molecules which are gases at room temperature. As you go down Group 7, the halogens become less reactive, have higher melting and boiling points. This is the exact opposite of alkali metals which get more reactive as you go down.
Halogens react with metals to produce salts which are held together by ionic bonds. The halogen becomes a halide ion with 1- charge. when they bond with metals. In order to write the salt formed, all you need to remember is that the net charge of the new compound formed must be 0. For example, sodium (an alkali metal) reacting with chlorine (a halogen) makes sodium chloride as seen above. Another example would be:
As we see, iron (a transition metal) reacts with bromine (a halogen) to make iron bromide. Remember that halogens are diatomic.
Halogens react covalently with non-metals to form compounds which all have a simple molecular structure:
Displacement reactions of Halogens
[edit | edit source]A more reactive halogen can displace a less reactive halogen from an aqueous solution of its salts. In simpler terms, the halogen further up the group will displace a halogen further down the group because reactivity decreases going down the group.
Notice how diatomic halogens lose their pairs when they react ionically. This is because they can obtain a full outershell by covalent bonding with the metal.
Quick Questions
[edit | edit source]What is the definition of Energy? |
The maximum amount of change possible in a system (object or group of objects) |
What are the 2 main types of energy store and what is the difference between them? |
Energy stores that relate to movement (Kinetic) and Potential energy stores which relate to the position of an object in space. |
What is energy measured in? |
Joules |
What is specific heat capacity? |
The amount of energy it takes to increase 1 Kg of a substance by 1 |
What is Power? |
The rate of energy transfer |
References
[edit | edit source]- ↑ http://www.bbc.co.uk/schools/gcsebitesize/science/edexcel_pre_2011/patterns/periodictablerev2.shtml
- ↑ http://www.bbc.co.uk/schools/gcsebitesize/science/edexcel_pre_2011/patterns/periodictablerev3.shtml
- ↑ http://www.bbc.co.uk/schools/gcsebitesize/science/edexcel_pre_2011/patterns/periodictablerev4.shtml
- ↑ https://courses.lumenlearning.com/cheminter/chapter/mendeleevs-periodic-table/
Carbon compounds
A Brief Introduction to Organic chemistry
[edit | edit source]Crude oil, hydrocarbons and alkanes
[edit | edit source]Crude oil is a finite resource found in rocks. It comes from the remains of ancient biomass (mainly plankton) that was buried in mud.
Crude oil is a mixture of a very large number of compounds. Most of the compounds are hydrocarbons, molecules made up of only hydrogen and carbon atoms only. There are different forms of hydrocarbons but the most common type are known as alkanes. Alkanes are a homologous series; they have the similar properties and follow a certain pattern of chemical formula. The general formula for alkanes is:
This is demonstrated by the first 4 alkanes in the series:
- Methane
- Ethane
- Propane
- Butane
We can also draw a structural diagram for alkanes by writing the carbon bonds in the center and filling all the other sides with hydrogen atoms. For example, the diagram for butane would look like this:

Fractional distillation and petrochemicals
[edit | edit source]Although crude oil is a mixture of many different hydrocarbons, we are able to separate hydrocarbons into fractions with compounds that have similar numbers of carbon atoms, by fractional distillation. The fractionating column has a temperature gradient, with the column being hottest at the bottom and coolest at the top. The longest hydrocarbons drain out first, and the shortest hydrocarbons, which have the lower boiling point, drain out at the top.
The fractions can be processed to produce fuels and feedstock for the petrochemical industry. Many of the fuels that we depend on come from crude oil such as petrol, diesel oil, kerosene, heavy fuel oil and petroleum gases. These are used to produce many useful materials such as solvents, lubricants, polymers and detergents.
All of this is thanks to the amazing ability of carbon atoms to form families of similar compounds (homologous series).
Properties of hydrocarbons
[edit | edit source]
Smaller hydrocarbons, by molecular size, have:
- higher viscosity (flow more easily)
- are more volatile
- have a lower boiling point
- and have a higher flammability (ignite more easily).
Longer hydrocarbons have:
- a higher boiling point
- lower viscosity
- not very volatile
- and low flammability.
These properties result in hydrocarbon fractions being used very differently. The longest hydrocarbon, bitumen, is used for road tarmac for example. Also, longer hydrocarbons are used as fuels for larger vehicles or systems such as heavy fuel oil being used for power stations and ships whilst shorter hydrocarbons such as petrol, is used as fuel for cars. The smallest hydrocarbon, liquified petroleum gas, is used as fuel for cooking and heating a house.
Combustion of hydrocarbons
[edit | edit source]Hydrocarbon fuels are burnt to release energy. This is known as combustion, and all combustion reactions are exothermic; they give out energy to the environment. When there is a good supply of oxygen (from the air) then combustion reactions are complete and follow this pattern:
This reaction provides the maximum amount of energy and is known as complete combustion. The following is a chemical equation for the complete combustion of ethane:
Combustion reactions are the only time in the chemistry course you can get away with half of a molecule, or alternatively you could double everything. In incomplete combustion carbon monoxide is produced instead of carbon dioxide:
Cracking and alkenes
[edit | edit source]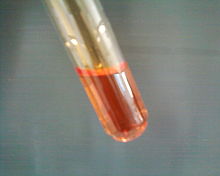
Cracking is when hydrocarbons are broken down into smaller molecules which are often more useful. Cracking is also an example of a thermal decomposition reaction where molecules are broken down by heating.
Catalytic cracking is when a catalyst is used to provide an alternative reaction pathway, lowering the temperature (around 500 Degrees Celsius) and pressure required for hydrocarbons to break down. The catalyst used is often zeolite which contains aluminium oxide and silicon oxide. On the other hand, steam (thermal) cracking does not use a catalyst and therefore requires a higher temperature (around 800 Degrees Celsius) and pressure. Catalytic cracking is much cheaper so is the preferred method for cracking hydrocarbons.
The products of cracking include smaller alkanes and another type of hydrocarbon known as alkenes:
Alkenes follow the same prefix (beginning bit of word) as alkanes however instead of -ane they have -ene. Ethene is the smallest alkene (there is no such thing as methene!).
The general formula for alkenes is:
If you recall, shorter alkene hydrocarbons are used for fuels of smaller vehicles such as cars, so smaller there is a far higher fuel demand for shorter hydrocarbons. Alkenes are used to produce polymers and as starting materials for many other chemicals.
Drawing alkenes
[edit | edit source]All alkenes have a carbon double covalent bond, , whilst alkanes only have single bonds. Here is the diagram for ethene:
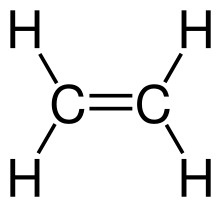
To draw larger alkenes:
- Cut off one side of the hydrogens from one side of the of ethene.
- Draw out the number of carbon atoms and bond each together such as for butene: (Remember that the left end should still have the 2 hydrogens)
- Fill all the sides with hydrogens except:
- There should only be 1 hydrogen to the other carbon in the double bond.
- There should be a hydrogen at the end.
This is what butene's displayed formula looks like:
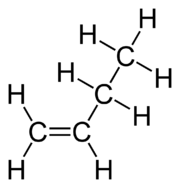
Test for alkenes
[edit | edit source]Alkenes are more reactive than alkanes, and therefore react with bromine water, an orange solution of bromine, by decolourising it into a colourless solution. Alkanes do not.
Quick Questions
[edit | edit source]What is the definition of Energy? |
The maximum amount of change possible in a system (object or group of objects) |
What are the 2 main types of energy store and what is the difference between them? |
Energy stores that relate to movement (Kinetic) and Potential energy stores which relate to the position of an object in space. |
What is energy measured in? |
Joules |
What is specific heat capacity? |
The amount of energy it takes to increase 1 Kg of a substance by 1 |
What is Power? |
The rate of energy transfer |
Further reading
[edit | edit source]
Reactions of alkenes and alcohols
Reactions of alkenes
[edit | edit source]Alkene molecules are unsaturated hydrocarbons because they contain 2 fewer hydrogen atoms than the alkane with the same number of carbon atoms and also have a carbon double bond instead of all single bonds. Alkanes are saturated hydrocarbons. You can distinguish saturated and unsaturated hydrocarbons via the bromine test.
Combustion of alkenes
[edit | edit source]In air there is not enough oxygen for the complete combustion of alkenes, which would produce only carbon dioxide and water (the same as the complete combustion of alkanes). Like other combustion reactions, this is an exothermic reaction; energy is given out. Instead they burn in air to produce smoky yellow flames:
The exact products formed depend on how much oxygen is present, so you could have the reaction with no carbon produced. Here is an example of the incomplete combustion of pentene (5 carbon):
Alternatively you could also the same reaction:
However the carbon allows you to balance it far more easily so is the preferred option.
Addition reactions
[edit | edit source]Alkenes react with hydrogen, water and the halogens, by the addition of atoms across the so that the double bond becomes a single bond.
Turning an alkene into an alkane (Hydrogenation)
[edit | edit source]An alkene can be turned back to an alkane when it reacts with hydrogen. This is an example with propene:
Remember that 2 hydrogen is the difference between the general formula for an alkene and that of an alkane. You also need to be able to draw the display formula:
![]() ![]() ![]() |
Propene + Hydrogen → Propane |
As we can see the carbon double bond, which is the functional group (the characteristic structure of a homologous series which gives it all of its special properties) of alkenes, is turned into a single bond with hydrogen. However, this reaction can only take place under the presence of a catalyst.
Reaction of halogens with alkenes
[edit | edit source]Halogens also remove the alkene functional group. Here is an example chemical formula with ethene and bromine:
A word equations helps to understand what is going on:
The di- refers to 2 bromine atoms in the compound and the -ane means that there is no longer any carbon double bonds; it has the same functional group as an alkane (all single bonds). The display formula would look like:
![]() ![]() |
Ethene + Bromine → Dibromoethane |
As we can see, this is almost the same as the hydrogenation, but instead we are using a halogen. This also explains why the bromine test works because the bromine atoms in the bromine water solution react to form a new compound whilst they would not react with an alkane.
Turning an alkene into an alcohol (add water)
[edit | edit source]Alcohols are a saturated hydrocarbon homologous series with a hydroxyl functional group (-OH). When an alkene reacts with water (requiring the presence of a catalyst) we can form an alcohol. Such as:
![]() ![]() ![]() |
But-1-ene + water → Butan-1-ol
|
We can easily work out the chemical formula for the alcohol as we know the functional group at the end (the -OH must be there) and all the carbons and hydrogens must balance. The product is called butanol with the -ol indicating it is an alcohol. The display formula is shown above: the only difference between an alkane structure and the alcohol structure is an oxygen atom as we can see.
Alcohols
[edit | edit source]Sometimes alcohols are written in a slightly different way to reflect there structure. For example:
is the chemical formula for ethanol, which can also be written as . The refers to one end of the compound, the refers to the carbon chain in the middle and the indicates the functional group. The general formula for an alcohol is:
All alcohols are soluble in water, with their solutions having a neutral pH. As a result of their solubility, they are commonly used as solvents in industry. Smaller alcohols are also used as fuels, for example ethanol is used as a spirit burner. It's most common use however is when it (ethanol) is found in alcoholic drinks.
Combustion of alcohols
[edit | edit source]Alcohols, which are flammable, undergo complete combustion (when burnt) in air:
Reaction with sodium
[edit | edit source]
Oxidation of alcohols
[edit | edit source]Alcohols can be oxidised (have oxygen added to them) to form carboxylic acids, these occur in different conditions to combustion.
Fermentation process
[edit | edit source]Aqueous solutions of ethanol are produced when sugar solutions are fermented using an enzyme in yeast:
This shows glucose, a simple sugar, being converted into ethanol and carbon dioxide. The reaction requires anaerobic (no oxygen) conditions, a fairly high temperature (37 Degrees Celsius) and a slightly acidic pH level.
Carboxylic acids
[edit | edit source]Carboxylic acids have the functional group and their names end in '-anoic acid'. Their structural diagram is similar to that of alcohols, although, a carbon-oxygen double bond is added. Here is a comparison of butanol and butanoic acid:
As we can see 2 hydrogen atoms have been removed and replaced with an oxygen double bond. The general formula for carboxylic acids is:
It is important to mention that for the nth term we use the carbons that have not been included in the functional group, so although butanoic acid has 4 carbon atoms, it has 3 which are not part of the functional group giving us the formula of:
Another way to write it would be:
Reaction with carbonates
[edit | edit source]Like any other acid, carboxylic acids react with a carbonate to produce a salt, water and carbon dioxide
Reaction with water
[edit | edit source]Carboxylic acids are able to dissolve in water. However, when they do, they ionise, releasing H+ ions which makes a weak acidic solution since they don't ionise completely.
Reaction with alcohols
[edit | edit source]Carboxylic acids react, with an acidic catalyst to make an ester (a new homologous series) and water.
As we can see from the chemical equation, the ester has the same at one end, as well as like a carboxylic acid. However, the hydrogen is removed and replaced with the alcohol's hydrocarbon chain, . It is no surprised then that the ester is called ethyl ethanoate (the alcohol is the -yl and the carboxylic acid is the -anoate). Drawing the ester can be tricky though:
The easiest way to draw an ester is to firstly draw the carboxylic acid without the OH. Then you add on the alcohol linking to the carbon and complete the alcohol structure, except from the hydrogen. These atoms bond to give the water that is also made from the reaction.
Quick Questions
[edit | edit source]What is the definition of Energy? |
The maximum amount of change possible in a system (object or group of objects) |
What are the 2 main types of energy store and what is the difference between them? |
Energy stores that relate to movement (Kinetic) and Potential energy stores which relate to the position of an object in space. |
What is energy measured in? |
Joules |
What is specific heat capacity? |
The amount of energy it takes to raise the temperature of 1 kg of a substance by 1 °C. |
What is Power? |
The rate of energy transfer |
Further reading
[edit | edit source]
Polymers
Addition Polymerisation
[edit | edit source]Alkenes can be used to make polymers by addition polymerisation. Here, unsaturated monomer molecules (alkenes) can react together, under pressure and with a catalyst to form a chain of repeating (poly) units with single carbon bonds.

Notice that the hydrogen atoms in the ethene compound are directly upwards or downwards. Also, we write a large n to show that we mean many (but an unspecified quantity of many). These form a long chain, which has bonds attaching either side, showing this.The polymer has exactly the same number of atoms as all of the monomers since that is the only product produced. So ethene monomers would make poly(ethene). For larger alkenes we cheat and write the same structure of ethene with an extra compound added on. For example with propene:

The polymer would look exactly the same as the above example with CH3 as opposed to a hydrogen.
Condensation polymerisation
[edit | edit source]Condensation polymerisation involves monomers with 2 different functional groups reacting together. This process often results in small molecules such as water being formed (thus they are called condensation reactions). The simplest polymers are produced from 2 different monomers with two of the same functional groups on each monomer:
The square in the diagram represents the carbon chains. The important thing are the endings, this is the chemical equation showing the important bits:
Notice that all of the hydrogen bonds with oxygen from the OH group of the dicarboxylic acid, we can get a functional group pattern:
Seems very similar?...
Amino acids
[edit | edit source]Amino acids have 2 different functional groups in a molecule - a basic amino group () and a carboxyl group (). The two groups can react in a condensation reaction to form a dipeptide:
Notice that the -OH and H form H2O and the rest remains the same (but goes upright) to form the polymer and water. Here is the chemical formula:
Polypeptides are proteins (chains of amino acids). Proteins contain different amino acids which give the protein different properties and instructions.
DNA
[edit | edit source]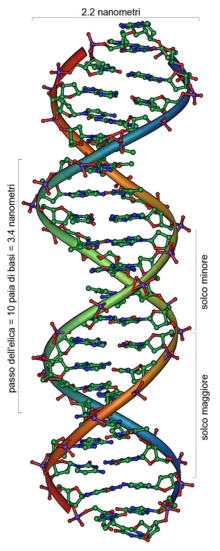
DNA (Deoxyribonucleic acid) is a large molecule essential for life. DNA encodes genetic instructions for the development and functioning of living organisms and viruses. Most DNA molecules are 2 polymer chains, made from four different monomers called nucleotides (A, T, C, G), in the form of a double helix. Other naturally occurring polymers include proteins, starch and cellulose.
Quick Questions
[edit | edit source]Further reading
[edit | edit source]
Identification of common gases
Test for hydrogen
[edit | edit source]Use a burning splint held at the open end of a test tube of gas. Hydrogen burns rapidly with a squeaky pop sound.
Test for oxygen
[edit | edit source]The test for oxygen uses a glowing splint inserted into a test tube of the gas. The splint relights in oxygen.
Test for carbon dioxide
[edit | edit source]An aqueous solution of calcium hydroxide, known as lime water. When carbon dioxide is shaken with or bubbled through limewater, the limewater turns cloudy.
Test for chlorine
[edit | edit source]When damp litmus paper is put into chlorine gas, the litmus paper is bleached and turns white.
Identification of ions
Flame tests can be used to identify some cations (positive ions).
Flame tests
[edit | edit source]Compound | colour of flame | Photo |
---|---|---|
Lithium | Crimson flame | ![]() |
Sodium | Yellow flame | ![]() |
Potassium | Lilac flame | ![]() |
Calcium | Orange-red flame | ![]() |
Copper | Green flame |
If a sample containing a mixture of ions is used, some flame colours can be masked.
Metal hydroxides
[edit | edit source]Sodium hydroxide solution can be used to identify some cations:
- Aluminium
- Calcium
- Magnesium
Ions form white precipitates when sodium hydroxide solution is added but only the aluminium hydroxide precipitate dissolves in excess sodium hydroxide solution.